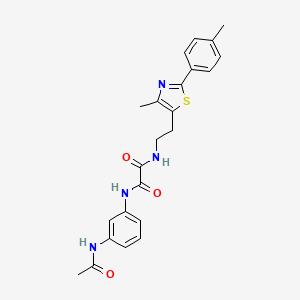
N1-(3-acetamidophenyl)-N2-(2-(4-methyl-2-(p-tolyl)thiazol-5-yl)ethyl)oxalamide
Overview
Description
Scientific Research Applications
S1QEL1.1 has a wide range of scientific research applications, including:
Chemistry: Used as a molecular probe to study electron transport and redox reactions in mitochondria.
Safety and Hazards
Future Directions
Mechanism of Action
Target of Action
S1QEL, also known as S1-QEL, N1-(3-acetamidophenyl)-N2-(2-(4-methyl-2-(p-tolyl)thiazol-5-yl)ethyl)oxalamide, S 1QEL, or S1QEL1.1, is a specific suppressor of superoxide production in the quinone-reaction site in mitochondrial complex I . This complex plays a crucial role in the electron transport chain, a series of protein complexes that transfer electrons through a membrane within mitochondria to drive the production of adenosine triphosphate (ATP), an essential carrier of energy in cells .
Mode of Action
The mode of action of S1QEL is unique and distinct from other known quinone-site inhibitors. The inhibitory potencies of S1QELs significantly vary depending on the direction of electron transfer (forward or reverse). S1QELs marginally suppress the specific chemical modification of Asp160 in the 49-kDa subunit, located deep in the quinone-binding pocket, by the tosyl chemistry reagent AL1 .
Biochemical Pathways
This indirect effect may be a prerequisite for S1QELs’ direction-dependent modulation of electron transfer .
Result of Action
The result of S1QEL’s action is the suppression of superoxide production during reverse electron transfer without significantly interfering with forward electron transfer . This, in turn, may be responsible for the suppression of excessive production of reactive oxygen species (ROS) from mitochondria, which is associated with a wide range of age-associated conditions .
Action Environment
The action environment of S1QEL is primarily within the mitochondria, specifically at the quinone-reaction site in mitochondrial complex I . The efficacy and stability of S1QEL could potentially be influenced by various environmental factors within the mitochondria, such as pH, temperature, and the presence of other molecules.
Biochemical Analysis
Biochemical Properties
S1QEL plays a crucial role in biochemical reactions by specifically inhibiting the production of superoxide at the quinone-reaction site in mitochondrial complex I . This inhibition is achieved without significantly affecting forward electron transfer, making it distinct from other quinone-site inhibitors . S1QEL interacts with the ND1 subunit of complex I, inducing structural changes that modulate quinone-redox reactions . This interaction is essential for its ability to suppress superoxide production during reverse electron transfer .
Cellular Effects
S1QEL has profound effects on various types of cells and cellular processes. By inhibiting superoxide production in mitochondria, S1QEL helps reduce oxidative stress, which is associated with a wide range of cellular dysfunctions and diseases . This compound influences cell signaling pathways, gene expression, and cellular metabolism by modulating the redox state within cells . The reduction in oxidative stress can lead to improved cell function and viability, making S1QEL a potential therapeutic agent for conditions related to mitochondrial dysfunction .
Molecular Mechanism
The molecular mechanism of S1QEL involves its binding to the ND1 subunit of mitochondrial complex I . This binding induces structural changes in the quinone-binding pocket, indirectly modulating quinone-redox reactions . Unlike other quinone-site inhibitors, S1QEL does not occupy the quinone- or inhibitor-binding pocket . Instead, it exerts its effects by inducing conformational changes that suppress superoxide production during reverse electron transfer without significantly interfering with forward electron transfer .
Temporal Effects in Laboratory Settings
In laboratory settings, the effects of S1QEL have been observed to change over time. Studies have shown that S1QEL remains stable and effective in inhibiting superoxide production over extended periods . Its long-term effects on cellular function are still being investigated.
Dosage Effects in Animal Models
The effects of S1QEL vary with different dosages in animal models. At lower doses, S1QEL effectively suppresses superoxide production without causing significant toxicity . At higher doses, some adverse effects have been observed, including potential toxicity and disruption of normal mitochondrial function . These findings highlight the importance of careful dosage optimization when using S1QEL in experimental settings .
Metabolic Pathways
S1QEL is involved in metabolic pathways related to mitochondrial function and oxidative stress . By inhibiting superoxide production at the quinone-reaction site in complex I, S1QEL affects the redox state of the cell and influences various metabolic processes . This compound interacts with enzymes and cofactors involved in mitochondrial electron transport, thereby modulating metabolic flux and metabolite levels .
Transport and Distribution
Within cells and tissues, S1QEL is transported and distributed primarily to mitochondria, where it exerts its effects . The compound interacts with mitochondrial transporters and binding proteins that facilitate its localization to the quinone-reaction site in complex I . This targeted distribution is crucial for its ability to specifically inhibit superoxide production without affecting other cellular processes .
Subcellular Localization
S1QEL is predominantly localized in the mitochondria, specifically at the quinone-reaction site in complex I . This subcellular localization is essential for its activity and function, as it allows S1QEL to effectively inhibit superoxide production during reverse electron transfer . The compound’s localization is facilitated by targeting signals and post-translational modifications that direct it to the appropriate mitochondrial compartments .
Preparation Methods
Synthetic Routes and Reaction Conditions
The synthesis of S1QEL1.1 involves several steps, including the use of Lawesson’s reagent, ethyl 2-chloroacetoacetate, and lithium aluminium hydride. The reactions are typically carried out under anhydrous conditions using dry solvents and standard syringe septum techniques. The synthetic route can be summarized as follows :
Step 1: Reaction of the starting material with Lawesson’s reagent in tetrahydrofuran under reflux conditions.
Step 2: Reaction with ethyl 2-chloroacetoacetate in ethanol under reflux conditions.
Step 3: Reduction using lithium aluminium hydride in tetrahydrofuran at 0°C to room temperature.
Step 4: Further reactions involving thionyl chloride, sodium cyanide, and other reagents to form the final product.
Industrial Production Methods
Industrial production methods for S1QEL1.1 are not well-documented, as it is primarily used in research settings. The synthesis is typically performed in laboratory-scale batches under controlled conditions to ensure purity and yield.
Chemical Reactions Analysis
Types of Reactions
S1QEL1.1 undergoes various chemical reactions, including:
Oxidation and Reduction: It can participate in redox reactions, particularly in the context of mitochondrial electron transport.
Substitution: It can undergo substitution reactions, especially in the presence of tosyl chemistry reagents.
Common Reagents and Conditions
Common reagents used in the reactions involving S1QEL1.1 include:
- Lawesson’s reagent
- Ethyl 2-chloroacetoacetate
- Lithium aluminium hydride
- Thionyl chloride
- Sodium cyanide
Major Products
The major products formed from these reactions are intermediates that lead to the final compound, S1QEL1.1. The specific products depend on the reaction conditions and reagents used.
Comparison with Similar Compounds
S1QEL1.1 is unique compared to other quinone-site inhibitors due to its direction-dependent modulation of electron transfer. Similar compounds include:
Piericidin A: Inhibits electron transfer in mitochondrial complex I but has different binding characteristics.
Rotenone: Another inhibitor of mitochondrial complex I, but it affects both forward and reverse electron transfer.
S1QEL1.1 stands out due to its specificity in suppressing superoxide production without altering oxidative phosphorylation, making it a valuable tool in mitochondrial research .
Properties
IUPAC Name |
N'-(3-acetamidophenyl)-N-[2-[4-methyl-2-(4-methylphenyl)-1,3-thiazol-5-yl]ethyl]oxamide | |
---|---|---|
Source | PubChem | |
URL | https://pubchem.ncbi.nlm.nih.gov | |
Description | Data deposited in or computed by PubChem | |
InChI |
InChI=1S/C23H24N4O3S/c1-14-7-9-17(10-8-14)23-25-15(2)20(31-23)11-12-24-21(29)22(30)27-19-6-4-5-18(13-19)26-16(3)28/h4-10,13H,11-12H2,1-3H3,(H,24,29)(H,26,28)(H,27,30) | |
Source | PubChem | |
URL | https://pubchem.ncbi.nlm.nih.gov | |
Description | Data deposited in or computed by PubChem | |
InChI Key |
BFNBJUBXXJKBFN-UHFFFAOYSA-N | |
Source | PubChem | |
URL | https://pubchem.ncbi.nlm.nih.gov | |
Description | Data deposited in or computed by PubChem | |
Canonical SMILES |
CC1=CC=C(C=C1)C2=NC(=C(S2)CCNC(=O)C(=O)NC3=CC=CC(=C3)NC(=O)C)C | |
Source | PubChem | |
URL | https://pubchem.ncbi.nlm.nih.gov | |
Description | Data deposited in or computed by PubChem | |
Molecular Formula |
C23H24N4O3S | |
Source | PubChem | |
URL | https://pubchem.ncbi.nlm.nih.gov | |
Description | Data deposited in or computed by PubChem | |
Molecular Weight |
436.5 g/mol | |
Source | PubChem | |
URL | https://pubchem.ncbi.nlm.nih.gov | |
Description | Data deposited in or computed by PubChem | |
Retrosynthesis Analysis
AI-Powered Synthesis Planning: Our tool employs the Template_relevance Pistachio, Template_relevance Bkms_metabolic, Template_relevance Pistachio_ringbreaker, Template_relevance Reaxys, Template_relevance Reaxys_biocatalysis model, leveraging a vast database of chemical reactions to predict feasible synthetic routes.
One-Step Synthesis Focus: Specifically designed for one-step synthesis, it provides concise and direct routes for your target compounds, streamlining the synthesis process.
Accurate Predictions: Utilizing the extensive PISTACHIO, BKMS_METABOLIC, PISTACHIO_RINGBREAKER, REAXYS, REAXYS_BIOCATALYSIS database, our tool offers high-accuracy predictions, reflecting the latest in chemical research and data.
Strategy Settings
Precursor scoring | Relevance Heuristic |
---|---|
Min. plausibility | 0.01 |
Model | Template_relevance |
Template Set | Pistachio/Bkms_metabolic/Pistachio_ringbreaker/Reaxys/Reaxys_biocatalysis |
Top-N result to add to graph | 6 |
Feasible Synthetic Routes
Disclaimer and Information on In-Vitro Research Products
Please be aware that all articles and product information presented on BenchChem are intended solely for informational purposes. The products available for purchase on BenchChem are specifically designed for in-vitro studies, which are conducted outside of living organisms. In-vitro studies, derived from the Latin term "in glass," involve experiments performed in controlled laboratory settings using cells or tissues. It is important to note that these products are not categorized as medicines or drugs, and they have not received approval from the FDA for the prevention, treatment, or cure of any medical condition, ailment, or disease. We must emphasize that any form of bodily introduction of these products into humans or animals is strictly prohibited by law. It is essential to adhere to these guidelines to ensure compliance with legal and ethical standards in research and experimentation.