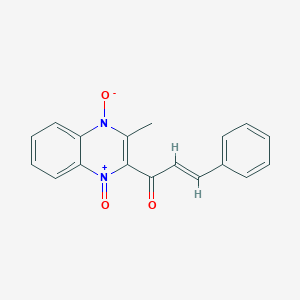
Quinocetone
Overview
Description
Quinocetone is a synthetic compound belonging to the quinoxaline-1,4-dioxide family. It is primarily used as an antibacterial agent in veterinary medicine, particularly in food-producing animals. This compound has been shown to possess broad-spectrum antibacterial activity, making it effective against a variety of bacterial pathogens .
Scientific Research Applications
Quinocetone has been extensively studied for its applications in various fields:
Chemistry: It serves as a model compound for studying the chemical behavior of quinoxaline derivatives.
Biology: this compound is used to investigate the mechanisms of bacterial resistance and the role of ROS in cellular damage.
Medicine: Research has focused on its potential use as an antibacterial agent in human medicine, although its use is currently limited to veterinary applications.
Industry: this compound is employed as a feed additive to promote growth and prevent infections in livestock
Mechanism of Action
Target of Action
Quinocetone, a member of the quinoxaline 1,4-di-N-oxides (QdNOs) family, primarily targets HepG2 cells . HepG2 cells are more sensitive to this compound compared to other cells . The compound also targets DNA, specifically guanine .
Mode of Action
This compound interacts with its targets by inducing the generation of superoxide anion (O2˙−) and hydroxyl radical (OH˙) during metabolism . These free radicals attack guanine and induce 8-hydroxy-deoxyguanine (8-OHdG) generation, causing DNA strand breakage . This process inhibits the activity of topoisomerase II (topo II) and alters the expression of genes such as PCNA, Gadd45, and topo II . This compound also causes mutations in the mtDNA genes COX1, COX3, and ATP6 .
Biochemical Pathways
This compound affects various biochemical pathways. It induces autophagy through the endoplasmic reticulum (ER) stress signaling pathway . This process involves the upregulation of immunoglobulin heavy chain binding protein (BiP), C/EBP homologous protein (CHOP), and the transcription factors BiP, HerpUD, and sec24D . Furthermore, this compound leads to the cleavage of ATF6, phosphorylation of MRLC (myosin regulatory light chain), and increased expression of death-associated protein kinase 1 (DAPK1) .
Pharmacokinetics
This compound exhibits complex pharmacokinetics characterized by rapid absorption and elimination in pigs and chickens . The absorption half-lives for pigs and chickens are approximately 0.46 hours and 0.51 hours, respectively, while the elimination half-lives are around 3.7 hours and 4.6 hours, respectively . This compound undergoes extensive metabolism prior to excretion, with multiple metabolites identified in various tissues and bodily fluids .
Result of Action
This compound triggers significant cytotoxicity and genotoxicity in vitro . It inhibits cell viability, induces DNA damage, causes cell cycle arrest, triggers reactive oxygen species (ROS) production, and disrupts mitochondrial function in cell lines such as HepG2 and Vero . This compound also induces apoptosis by activating the p38/Nrf2/HO-1 pathway and inhibiting the ROS/mitochondrial apoptotic pathway .
Action Environment
The action of this compound is influenced by various environmental factors. For instance, the compound’s cytotoxicity and genotoxicity can be attenuated by quercetin, a plant flavonol from the flavonoid group of polyphenols . Quercetin improves this compound-induced cytotoxicity and oxidative damage in human L02 and HepG2 cells . It also inhibits this compound-induced loss of mitochondrial membrane potential and increases in the expression of the CytC protein and the Bax/Bcl-2 ratio .
Safety and Hazards
Future Directions
Quercetin has been found to attenuate Quinocetone-induced cell apoptosis in vitro by activating the P38/Nrf2/HO-1 pathway and inhibiting the ROS/mitochondrial apoptotic pathway . This suggests that quercetin may be a promising candidate for preventing QdNOs-induced cytotoxicity in humans or animals .
Biochemical Analysis
Biochemical Properties
Quinocetone plays a significant role in biochemical reactions, particularly due to its interaction with various enzymes, proteins, and other biomolecules. It has been found to induce the generation of reactive oxygen species (ROS) such as superoxide anion (O2˙−) and hydroxyl radical (OH˙) during metabolism . These ROS can attack guanine bases in DNA, leading to the formation of 8-hydroxy-deoxyguanine (8-OHdG), which causes DNA strand breakage . This compound also inhibits the activity of topoisomerase II, an enzyme crucial for DNA replication and repair . Additionally, it affects the expression of genes such as PCNA, Gadd45, and topoisomerase II, further influencing cellular processes .
Cellular Effects
This compound exerts various effects on different cell types and cellular processes. In HepG2 cells, it has been shown to induce significant cytotoxicity and genotoxicity . This compound influences cell function by generating ROS, which can lead to oxidative stress and DNA damage . It also affects cell signaling pathways and gene expression, altering the expression of genes involved in DNA repair and apoptosis . Furthermore, this compound impacts cellular metabolism by affecting the mitochondrial respiratory chain, leading to increased production of ROS and potential mitochondrial dysfunction .
Molecular Mechanism
At the molecular level, this compound exerts its effects through several mechanisms. It binds electrostatically to DNA, affecting the dissociation of topoisomerase II from DNA and impacting DNA replication . This compound also induces the generation of ROS during metabolism, which can cause oxidative damage to DNA and other cellular components . Additionally, it affects the expression of genes involved in DNA repair and apoptosis, further contributing to its genotoxic effects .
Temporal Effects in Laboratory Settings
In laboratory settings, the effects of this compound change over time. This compound is known to be relatively stable, but its degradation products can also exert biological effects . Long-term exposure to this compound has been shown to cause persistent oxidative stress and DNA damage in cells . In vitro studies have demonstrated that this compound can induce apoptosis through both mitochondria-dependent and mitochondria-independent pathways .
Dosage Effects in Animal Models
The effects of this compound vary with different dosages in animal models. At low doses, this compound promotes growth and prevents bacterial infections in food-producing animals . At high doses, it can cause toxic effects, including oxidative stress, DNA damage, and potential carcinogenicity . Threshold effects have been observed, where the genotoxic effects of this compound become more pronounced at higher concentrations .
Metabolic Pathways
This compound is involved in several metabolic pathways, primarily through its interaction with enzymes and cofactors. During metabolism, this compound generates ROS, which can lead to oxidative damage to cellular components . It also affects the activity of enzymes involved in DNA repair and apoptosis, further contributing to its genotoxic effects . Additionally, this compound influences metabolic flux and metabolite levels, impacting cellular metabolism .
Transport and Distribution
This compound is transported and distributed within cells and tissues through various mechanisms. It interacts with transporters and binding proteins that facilitate its uptake and distribution . This compound can accumulate in specific tissues, leading to localized effects on cellular function . Its distribution within cells can also affect its localization and accumulation, influencing its biological activity .
Subcellular Localization
The subcellular localization of this compound plays a crucial role in its activity and function. This compound can be targeted to specific compartments or organelles through targeting signals or post-translational modifications . Its localization within cells can affect its interaction with biomolecules and its overall biological activity . This compound’s effects on subcellular compartments, such as the mitochondria, can lead to mitochondrial dysfunction and increased ROS production .
Preparation Methods
Synthetic Routes and Reaction Conditions: The synthesis of quinocetone typically involves the reaction of benzofurazan, acetylacetone, and benzaldehyde. The process begins with the formation of an intermediate compound, which is then subjected to further reactions to yield this compound .
Industrial Production Methods: In industrial settings, the production of this compound involves a series of well-controlled chemical reactions. The process is optimized to ensure high yield and purity of the final product. The use of advanced techniques such as high-performance liquid chromatography (HPLC) is common to monitor the quality of this compound during production .
Chemical Reactions Analysis
Types of Reactions: Quinocetone undergoes various chemical reactions, including oxidation, reduction, and substitution. These reactions are essential for its antibacterial activity and other biological effects .
Common Reagents and Conditions:
Oxidation: this compound can be oxidized in the presence of reactive oxygen species (ROS), leading to the formation of reactive intermediates.
Reduction: The reduction of this compound involves the use of reducing agents such as sodium dithionite.
Substitution: Substitution reactions of this compound often involve nucleophilic reagents that replace specific functional groups on the molecule.
Major Products Formed: The major products formed from these reactions include various quinoxaline derivatives, which retain the antibacterial properties of the parent compound .
Comparison with Similar Compounds
- Carbadox
- Olaquindox
- Mequindox
These compounds share a similar core structure but differ in their specific functional groups and biological activities .
Properties
IUPAC Name |
(E)-1-(3-methyl-4-oxido-1-oxoquinoxalin-1-ium-2-yl)-3-phenylprop-2-en-1-one | |
---|---|---|
Source | PubChem | |
URL | https://pubchem.ncbi.nlm.nih.gov | |
Description | Data deposited in or computed by PubChem | |
InChI |
InChI=1S/C18H14N2O3/c1-13-18(17(21)12-11-14-7-3-2-4-8-14)20(23)16-10-6-5-9-15(16)19(13)22/h2-12H,1H3/b12-11+ | |
Source | PubChem | |
URL | https://pubchem.ncbi.nlm.nih.gov | |
Description | Data deposited in or computed by PubChem | |
InChI Key |
IOKWXGMNRWVQHX-VAWYXSNFSA-N | |
Source | PubChem | |
URL | https://pubchem.ncbi.nlm.nih.gov | |
Description | Data deposited in or computed by PubChem | |
Canonical SMILES |
CC1=C([N+](=O)C2=CC=CC=C2N1[O-])C(=O)C=CC3=CC=CC=C3 | |
Source | PubChem | |
URL | https://pubchem.ncbi.nlm.nih.gov | |
Description | Data deposited in or computed by PubChem | |
Isomeric SMILES |
CC1=C([N+](=O)C2=CC=CC=C2N1[O-])C(=O)/C=C/C3=CC=CC=C3 | |
Source | PubChem | |
URL | https://pubchem.ncbi.nlm.nih.gov | |
Description | Data deposited in or computed by PubChem | |
Molecular Formula |
C18H14N2O3 | |
Source | PubChem | |
URL | https://pubchem.ncbi.nlm.nih.gov | |
Description | Data deposited in or computed by PubChem | |
Molecular Weight |
306.3 g/mol | |
Source | PubChem | |
URL | https://pubchem.ncbi.nlm.nih.gov | |
Description | Data deposited in or computed by PubChem | |
Retrosynthesis Analysis
AI-Powered Synthesis Planning: Our tool employs the Template_relevance Pistachio, Template_relevance Bkms_metabolic, Template_relevance Pistachio_ringbreaker, Template_relevance Reaxys, Template_relevance Reaxys_biocatalysis model, leveraging a vast database of chemical reactions to predict feasible synthetic routes.
One-Step Synthesis Focus: Specifically designed for one-step synthesis, it provides concise and direct routes for your target compounds, streamlining the synthesis process.
Accurate Predictions: Utilizing the extensive PISTACHIO, BKMS_METABOLIC, PISTACHIO_RINGBREAKER, REAXYS, REAXYS_BIOCATALYSIS database, our tool offers high-accuracy predictions, reflecting the latest in chemical research and data.
Strategy Settings
Precursor scoring | Relevance Heuristic |
---|---|
Min. plausibility | 0.01 |
Model | Template_relevance |
Template Set | Pistachio/Bkms_metabolic/Pistachio_ringbreaker/Reaxys/Reaxys_biocatalysis |
Top-N result to add to graph | 6 |
Feasible Synthetic Routes
Q1: What is the primary mechanism of action of Quinocetone?
A1: While the precise mechanism of action remains unclear, research suggests that this compound may exert its antimicrobial effects by interfering with bacterial DNA synthesis. [] Further research is needed to elucidate the exact molecular targets and downstream effects.
Q2: What is the molecular formula and weight of this compound?
A2: this compound has the molecular formula C18H14N2O3 and a molecular weight of 306.31 g/mol. []
Q3: Are there any spectroscopic data available for this compound?
A3: Yes, studies utilize various spectroscopic techniques, including 1H-NMR, 13C-NMR, and mass spectrometry, to characterize this compound and its metabolites. [, ]
Q4: Is there information on the stability of this compound under different conditions?
A4: While specific stability data are limited in the provided research, one study mentions improved solubility of this compound sulfonate derivatives. [] Further research is needed to comprehensively assess its stability under various conditions like temperature, pH, and light exposure.
Q5: How is this compound absorbed, distributed, metabolized, and excreted (ADME) in different species?
A5: Studies demonstrate that this compound is rapidly absorbed and widely distributed in various species, including pigs, chickens, carp, and ducks. [, , , , ] The liver appears to be the primary site of metabolism, with major metabolites including 1-desoxythis compound, di-desoxythis compound, and 3-methylquinoxaline-2-carboxylic acid. [, , , , , ] Excretion pathways vary between species.
Q6: What is the bioavailability of this compound after oral administration?
A6: Bioavailability varies depending on the species. In chickens, it is relatively low at 21.47%. [] In ducks, the bioavailability was determined to be 38.43%. []
Q7: Has this compound demonstrated growth-promoting effects in animal studies?
A7: Yes, several studies report that dietary this compound supplementation enhances growth performance in broiler chickens, exhibiting comparable or even superior effects to Olaquindox. [, , ]
Q8: What is the safety profile of this compound?
A8: While considered relatively safe, a subchronic toxicity study in rats identified adverse effects at high doses (820 mg/kg bw/day), including mortality, reduced body weight, and altered organ weights. [] The no-observed-effect level (NOEL) in this study was determined to be 32.8 mg/kg bw/day. []
Q9: What analytical techniques are employed to detect and quantify this compound and its metabolites?
A9: High-performance liquid chromatography (HPLC) coupled with ultraviolet (UV) detection or tandem mass spectrometry (MS/MS) are the predominant methods for analyzing this compound residues in various matrices. [, , , , , , , , , ]
Q10: How do structural modifications affect the activity of this compound?
A10: Research on this compound derivatives, specifically the introduction of hydroxyl groups, reveals a significant impact on solubility. [] The position of the hydroxyl group influences both solubility and antibacterial activity, highlighting the importance of structural modifications in optimizing drug properties. []
Disclaimer and Information on In-Vitro Research Products
Please be aware that all articles and product information presented on BenchChem are intended solely for informational purposes. The products available for purchase on BenchChem are specifically designed for in-vitro studies, which are conducted outside of living organisms. In-vitro studies, derived from the Latin term "in glass," involve experiments performed in controlled laboratory settings using cells or tissues. It is important to note that these products are not categorized as medicines or drugs, and they have not received approval from the FDA for the prevention, treatment, or cure of any medical condition, ailment, or disease. We must emphasize that any form of bodily introduction of these products into humans or animals is strictly prohibited by law. It is essential to adhere to these guidelines to ensure compliance with legal and ethical standards in research and experimentation.