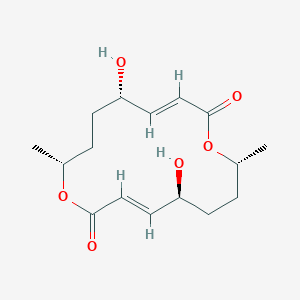
Pyrenophorol
Overview
Description
Pyrenophorol is a natural product isolated from the culture filtrates of the phytopathogenic fungus Stemphylium radicinum (C.M.I. 105654) Its chemical formula is C9H12O3
Mechanism of Action
Target of Action
Pyrenophorol is a macrodiolide, a class of natural products isolated from various fungi It’s known that macrodiolides like this compound exhibit various bioactivities, including strong antifungal, antihelmintic, and phytotoxic activity .
Mode of Action
A unique flavin enzyme, pyle, has been identified to catalyze the isomerization of the 4-alcohol-2,3-unsaturated part within the dilactone scaffold of this compound, forming a 1,4-diketone . This suggests that this compound may interact with its targets through this enzymatically catalyzed isomerization.
Biochemical Pathways
Given its antifungal and phytotoxic activities, it can be inferred that this compound likely interferes with essential biochemical pathways in fungi and plants, leading to their inhibition or death .
Pharmacokinetics
As a general rule, these properties play a crucial role in determining the bioavailability and overall effectiveness of a compound
Result of Action
Given its antifungal and phytotoxic activities, it can be inferred that this compound likely induces cellular damage or dysfunction in fungi and plants, leading to their inhibition or death .
Action Environment
Environmental factors can significantly influence the action, efficacy, and stability of a compound. For instance, the toxicity of this compound varies among different aquatic organisms, suggesting that its action can be influenced by the specific characteristics of the organism and its environment
Biochemical Analysis
Biochemical Properties
Pyrenophorol interacts with various enzymes and proteins in biochemical reactions. It is known to inhibit seed germination but once the seed is germinated, this compound enhances root development but causes abnormal chlorophyll retention in leaf sections . It is soluble in ethanol, methanol, DMF, and DMSO .
Cellular Effects
This compound has significant effects on various types of cells and cellular processes. It has been found to inhibit human topoisomerase II α when used at concentrations of 75 and 100 μM . It is active against S. cerevisiae (MIC = 4 μM) and M. violaceum . This compound induces leaf necrosis and chlorophyll retention in wild oats .
Molecular Mechanism
The molecular mechanism of this compound’s action involves its interaction with biomolecules and its influence on gene expression. A unique flavoenzyme, PylE, has been identified that can catalyze the isomerization of the diolide scaffold’s 4-alcohol-2,3-unsaturated part, thereby forming a 1,4-diketone . Further understanding of PylE’s catalytic mechanism has been gained through mutagenesis experiments combined with molecular docking .
Temporal Effects in Laboratory Settings
It is known that this compound strongly inhibits bacterial bioluminescence, and this effect is almost complete within 5 minutes of exposure .
Metabolic Pathways
It is known that this compound is a product of a biosynthetic gene cluster responsible for producing C2 asymmetric 16-membered dilactones . The biosynthetic pathway of this compound dilactone has been elucidated .
Preparation Methods
The synthetic routes for Pyrenophorol are not extensively documented, but it can be obtained from the culture filtrates of S. radicinum. Industrial production methods are limited, likely due to its low yield.
Chemical Reactions Analysis
Pyrenophorol undergoes various chemical reactions, including oxidation, reduction, and substitution. Common reagents and conditions used in these reactions are not well-established. further research is needed to explore its reactivity fully. Major products resulting from these reactions remain an area of investigation.
Scientific Research Applications
Pyrenophorol’s applications span several scientific fields:
Chemistry: Researchers study its reactivity and potential as a synthetic intermediate.
Biology: Investigations focus on its biological activity, including antimicrobial properties.
Medicine: Its potential therapeutic effects are being explored.
Industry: Although limited, this compound may find applications in agrochemicals or pharmaceuticals.
Comparison with Similar Compounds
While Pyrenophorol is unique, it shares structural features with other natural products. its distinct properties set it apart. Similar compounds include :
- Alternaria-derived metabolites
- Other fungal secondary metabolites
Properties
IUPAC Name |
(3E,5S,8R,11E,13S,16R)-5,13-dihydroxy-8,16-dimethyl-1,9-dioxacyclohexadeca-3,11-diene-2,10-dione | |
---|---|---|
Source | PubChem | |
URL | https://pubchem.ncbi.nlm.nih.gov | |
Description | Data deposited in or computed by PubChem | |
InChI |
InChI=1S/C16H24O6/c1-11-3-5-13(17)8-10-16(20)22-12(2)4-6-14(18)7-9-15(19)21-11/h7-14,17-18H,3-6H2,1-2H3/b9-7+,10-8+/t11-,12-,13+,14+/m1/s1 | |
Source | PubChem | |
URL | https://pubchem.ncbi.nlm.nih.gov | |
Description | Data deposited in or computed by PubChem | |
InChI Key |
RBQNDQOKFICJGL-UTBFYLPBSA-N | |
Source | PubChem | |
URL | https://pubchem.ncbi.nlm.nih.gov | |
Description | Data deposited in or computed by PubChem | |
Canonical SMILES |
CC1CCC(C=CC(=O)OC(CCC(C=CC(=O)O1)O)C)O | |
Source | PubChem | |
URL | https://pubchem.ncbi.nlm.nih.gov | |
Description | Data deposited in or computed by PubChem | |
Isomeric SMILES |
C[C@H]1OC(=O)/C=C/[C@H](CC[C@H](OC(=O)/C=C/[C@H](CC1)O)C)O | |
Source | PubChem | |
URL | https://pubchem.ncbi.nlm.nih.gov | |
Description | Data deposited in or computed by PubChem | |
Molecular Formula |
C16H24O6 | |
Source | PubChem | |
URL | https://pubchem.ncbi.nlm.nih.gov | |
Description | Data deposited in or computed by PubChem | |
DSSTOX Substance ID |
DTXSID30448991 | |
Record name | Pyrenophorol | |
Source | EPA DSSTox | |
URL | https://comptox.epa.gov/dashboard/DTXSID30448991 | |
Description | DSSTox provides a high quality public chemistry resource for supporting improved predictive toxicology. | |
Molecular Weight |
312.36 g/mol | |
Source | PubChem | |
URL | https://pubchem.ncbi.nlm.nih.gov | |
Description | Data deposited in or computed by PubChem | |
CAS No. |
22248-41-5 | |
Record name | Pyrenophorol | |
Source | EPA DSSTox | |
URL | https://comptox.epa.gov/dashboard/DTXSID30448991 | |
Description | DSSTox provides a high quality public chemistry resource for supporting improved predictive toxicology. | |
Retrosynthesis Analysis
AI-Powered Synthesis Planning: Our tool employs the Template_relevance Pistachio, Template_relevance Bkms_metabolic, Template_relevance Pistachio_ringbreaker, Template_relevance Reaxys, Template_relevance Reaxys_biocatalysis model, leveraging a vast database of chemical reactions to predict feasible synthetic routes.
One-Step Synthesis Focus: Specifically designed for one-step synthesis, it provides concise and direct routes for your target compounds, streamlining the synthesis process.
Accurate Predictions: Utilizing the extensive PISTACHIO, BKMS_METABOLIC, PISTACHIO_RINGBREAKER, REAXYS, REAXYS_BIOCATALYSIS database, our tool offers high-accuracy predictions, reflecting the latest in chemical research and data.
Strategy Settings
Precursor scoring | Relevance Heuristic |
---|---|
Min. plausibility | 0.01 |
Model | Template_relevance |
Template Set | Pistachio/Bkms_metabolic/Pistachio_ringbreaker/Reaxys/Reaxys_biocatalysis |
Top-N result to add to graph | 6 |
Feasible Synthetic Routes
Disclaimer and Information on In-Vitro Research Products
Please be aware that all articles and product information presented on BenchChem are intended solely for informational purposes. The products available for purchase on BenchChem are specifically designed for in-vitro studies, which are conducted outside of living organisms. In-vitro studies, derived from the Latin term "in glass," involve experiments performed in controlled laboratory settings using cells or tissues. It is important to note that these products are not categorized as medicines or drugs, and they have not received approval from the FDA for the prevention, treatment, or cure of any medical condition, ailment, or disease. We must emphasize that any form of bodily introduction of these products into humans or animals is strictly prohibited by law. It is essential to adhere to these guidelines to ensure compliance with legal and ethical standards in research and experimentation.