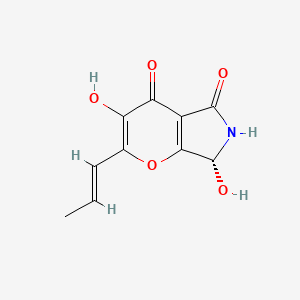
Pyranonigrin A
Overview
Description
Pyranonigrin A is a member of the pyranonigrin family, which are antioxidative compounds produced by the fungus Aspergillus niger. These compounds feature a unique pyrano[2,3-c]pyrrole bicyclic skeleton, which is rarely found in nature . This compound has been shown to have significant activity as a 1,1-diphenyl-2-picrylhydrazyl radical scavenging reagent .
Mechanism of Action
Target of Action
Pyranonigrin A is a secondary metabolite produced by Aspergillus niger . It has been shown to have a potent 2,2-diphenyl-1-picrylhydrazyl (DPPH) radical scavenging activity . Therefore, the primary target of this compound is the DPPH radical, a common proxy for reactive oxygen species (ROS) that can cause oxidative stress in cells.
Mode of Action
This compound interacts with DPPH radicals, neutralizing them and thereby reducing oxidative stress . This interaction results in the stabilization of cellular processes that could be disrupted by the presence of ROS.
Biochemical Pathways
The biosynthesis of this compound involves a polyketide synthase (PKS) and nonribosomal peptide synthetase (NRPS) hybrid enzyme . Recent isotope labeling studies showed that the backbone of this compound is derived from four units of acetate and one unit of glycine . The PKS module functions iteratively to produce a polyketide chain with mixed degrees of α-methylation and β-reduction during each chain elongation cycle . The NRPS module captures the completed polyketide chain in the form of a thioester and condenses with a specifically activated amino acid (by the adenylation domain) to form an amide bond .
Result of Action
The primary result of this compound’s action is the reduction of oxidative stress in cells . By scavenging DPPH radicals, this compound helps to maintain the balance of ROS in cells, preventing damage to cellular components such as proteins, lipids, and DNA.
Action Environment
The action of this compound is influenced by the environment in which it is present. For example, the production of this compound by Aspergillus niger is likely influenced by the growth conditions of the fungus . Additionally, the efficacy of this compound in scavenging DPPH radicals may be influenced by the concentration of ROS in the cell and the presence of other antioxidants.
Biochemical Analysis
Biochemical Properties
Pyranonigrin A plays a significant role in biochemical reactions, primarily due to its antioxidative properties. It interacts with various enzymes, proteins, and other biomolecules to exert its effects. One of the key interactions involves the enzyme polyketide synthase, which is part of the biosynthetic pathway of this compound . Additionally, this compound has been shown to interact with nonribosomal peptide synthetase, which aids in the formation of its unique structure . These interactions are crucial for the compound’s ability to scavenge free radicals and protect cells from oxidative stress.
Cellular Effects
This compound influences various types of cells and cellular processes. It has been observed to affect cell signaling pathways, gene expression, and cellular metabolism. For instance, this compound can modulate the expression of genes involved in oxidative stress response, thereby enhancing the cell’s ability to cope with reactive oxygen species . Additionally, this compound has been shown to impact cellular metabolism by interacting with key metabolic enzymes, leading to changes in metabolic flux and metabolite levels .
Molecular Mechanism
The mechanism of action of this compound involves several molecular interactions. At the molecular level, this compound binds to specific biomolecules, including enzymes and proteins, to exert its effects. One of the primary mechanisms is the inhibition of oxidative stress-related enzymes, which helps in reducing the levels of reactive oxygen species within the cell . Furthermore, this compound can activate certain transcription factors, leading to changes in gene expression that promote cellular defense mechanisms .
Temporal Effects in Laboratory Settings
In laboratory settings, the effects of this compound have been studied over time to understand its stability, degradation, and long-term impact on cellular function. This compound has been found to be relatively stable under various conditions, maintaining its antioxidative properties over extended periods . Prolonged exposure to certain environmental factors, such as UV radiation, can lead to its degradation . Long-term studies have shown that this compound can have sustained effects on cellular function, particularly in enhancing the cell’s ability to manage oxidative stress .
Dosage Effects in Animal Models
The effects of this compound vary with different dosages in animal models. At lower doses, this compound has been shown to provide antioxidative benefits without any adverse effects . At higher doses, there may be threshold effects, including potential toxicity and adverse reactions . It is crucial to determine the optimal dosage to maximize the therapeutic benefits of this compound while minimizing any potential risks.
Metabolic Pathways
This compound is involved in several metabolic pathways, interacting with various enzymes and cofactors. The biosynthesis of this compound involves the enzyme polyketide synthase, which catalyzes the formation of its unique structure . Additionally, this compound interacts with nonribosomal peptide synthetase, which plays a role in its assembly . These interactions are essential for the production and function of this compound within the cell.
Transport and Distribution
Within cells and tissues, this compound is transported and distributed through specific transporters and binding proteins. These transporters facilitate the movement of this compound to its target sites, ensuring its proper localization and accumulation . The distribution of this compound within the cell is crucial for its antioxidative function, as it needs to be present in areas where oxidative stress is prevalent .
Subcellular Localization
This compound exhibits specific subcellular localization, which is essential for its activity and function. It is often directed to compartments or organelles where oxidative stress is most significant, such as mitochondria . This localization is facilitated by targeting signals and post-translational modifications that guide this compound to its appropriate sites within the cell . The subcellular localization of this compound is critical for its ability to protect cells from oxidative damage.
Preparation Methods
Synthetic Routes and Reaction Conditions: The biosynthesis of pyranonigrin A involves a polyketide synthase and nonribosomal peptide synthetase hybrid enzyme. The backbone of this compound is derived from four units of acetate and one unit of glycine . The polyketide synthase module functions iteratively to produce a polyketide chain, which is then captured by the nonribosomal peptide synthetase module to form an amide bond .
Industrial Production Methods: Industrial production of this compound can be achieved through heterologous expression of the biosynthetic gene cluster in a suitable host organism.
Chemical Reactions Analysis
Types of Reactions: Pyranonigrin A undergoes various chemical reactions, including oxidation, reduction, and substitution reactions. These reactions are facilitated by the presence of functional groups such as hydroxyl and carbonyl groups in its structure .
Common Reagents and Conditions: Common reagents used in the reactions of this compound include oxidizing agents like hydrogen peroxide and reducing agents like sodium borohydride. The reactions are typically carried out under mild conditions to preserve the integrity of the pyrano[2,3-c]pyrrole skeleton .
Major Products Formed: The major products formed from the reactions of this compound include oxidized and reduced derivatives, which retain the core pyrano[2,3-c]pyrrole structure .
Scientific Research Applications
Pyranonigrin A has a wide range of scientific research applications due to its antioxidative properties. In chemistry, it is used as a radical scavenging reagent. In biology, it is studied for its potential role in protecting cells from oxidative stress. In medicine, this compound is being investigated for its potential therapeutic applications, including its use as an antioxidant in the treatment of diseases related to oxidative stress .
Comparison with Similar Compounds
Pyranonigrin A is unique among the pyranonigrin family due to its specific structure and antioxidative properties. Similar compounds include pyranonigrin E and pyranonigrin S, which also possess antioxidative properties but differ in their structural features and biosynthetic pathways . Pyranonigrin E, for example, has a different alkyl chain length and amino acid origin compared to this compound .
Properties
IUPAC Name |
(7R)-3,7-dihydroxy-2-[(E)-prop-1-enyl]-6,7-dihydropyrano[2,3-c]pyrrole-4,5-dione | |
---|---|---|
Source | PubChem | |
URL | https://pubchem.ncbi.nlm.nih.gov | |
Description | Data deposited in or computed by PubChem | |
InChI |
InChI=1S/C10H9NO5/c1-2-3-4-6(12)7(13)5-8(16-4)10(15)11-9(5)14/h2-3,10,12,15H,1H3,(H,11,14)/b3-2+/t10-/m1/s1 | |
Source | PubChem | |
URL | https://pubchem.ncbi.nlm.nih.gov | |
Description | Data deposited in or computed by PubChem | |
InChI Key |
OALBJWDVDNROSF-VMZHVLLKSA-N | |
Source | PubChem | |
URL | https://pubchem.ncbi.nlm.nih.gov | |
Description | Data deposited in or computed by PubChem | |
Canonical SMILES |
CC=CC1=C(C(=O)C2=C(O1)C(NC2=O)O)O | |
Source | PubChem | |
URL | https://pubchem.ncbi.nlm.nih.gov | |
Description | Data deposited in or computed by PubChem | |
Isomeric SMILES |
C/C=C/C1=C(C(=O)C2=C(O1)[C@H](NC2=O)O)O | |
Source | PubChem | |
URL | https://pubchem.ncbi.nlm.nih.gov | |
Description | Data deposited in or computed by PubChem | |
Molecular Formula |
C10H9NO5 | |
Source | PubChem | |
URL | https://pubchem.ncbi.nlm.nih.gov | |
Description | Data deposited in or computed by PubChem | |
DSSTOX Substance ID |
DTXSID501017485 | |
Record name | Pyranonigrin A | |
Source | EPA DSSTox | |
URL | https://comptox.epa.gov/dashboard/DTXSID501017485 | |
Description | DSSTox provides a high quality public chemistry resource for supporting improved predictive toxicology. | |
Molecular Weight |
223.18 g/mol | |
Source | PubChem | |
URL | https://pubchem.ncbi.nlm.nih.gov | |
Description | Data deposited in or computed by PubChem | |
CAS No. |
773855-65-5 | |
Record name | (7R)-6,7-Dihydro-3,7-dihydroxy-2-(1E)-1-propen-1-ylpyrano[2,3-c]pyrrole-4,5-dione | |
Source | CAS Common Chemistry | |
URL | https://commonchemistry.cas.org/detail?cas_rn=773855-65-5 | |
Description | CAS Common Chemistry is an open community resource for accessing chemical information. Nearly 500,000 chemical substances from CAS REGISTRY cover areas of community interest, including common and frequently regulated chemicals, and those relevant to high school and undergraduate chemistry classes. This chemical information, curated by our expert scientists, is provided in alignment with our mission as a division of the American Chemical Society. | |
Explanation | The data from CAS Common Chemistry is provided under a CC-BY-NC 4.0 license, unless otherwise stated. | |
Record name | Pyranonigrin A | |
Source | EPA DSSTox | |
URL | https://comptox.epa.gov/dashboard/DTXSID501017485 | |
Description | DSSTox provides a high quality public chemistry resource for supporting improved predictive toxicology. | |
Retrosynthesis Analysis
AI-Powered Synthesis Planning: Our tool employs the Template_relevance Pistachio, Template_relevance Bkms_metabolic, Template_relevance Pistachio_ringbreaker, Template_relevance Reaxys, Template_relevance Reaxys_biocatalysis model, leveraging a vast database of chemical reactions to predict feasible synthetic routes.
One-Step Synthesis Focus: Specifically designed for one-step synthesis, it provides concise and direct routes for your target compounds, streamlining the synthesis process.
Accurate Predictions: Utilizing the extensive PISTACHIO, BKMS_METABOLIC, PISTACHIO_RINGBREAKER, REAXYS, REAXYS_BIOCATALYSIS database, our tool offers high-accuracy predictions, reflecting the latest in chemical research and data.
Strategy Settings
Precursor scoring | Relevance Heuristic |
---|---|
Min. plausibility | 0.01 |
Model | Template_relevance |
Template Set | Pistachio/Bkms_metabolic/Pistachio_ringbreaker/Reaxys/Reaxys_biocatalysis |
Top-N result to add to graph | 6 |
Feasible Synthetic Routes
Q1: What is Pyranonigrin A and where is it found?
A1: this compound is a secondary metabolite belonging to the pyranonigrin family, characterized by a unique pyrano[2,3-c]pyrrole bicyclic skeleton. [] It is primarily produced by various species of Aspergillus, a genus of fungi found globally in diverse environments, including soil, plants, and even the International Space Station. [, , , , , , , ] this compound has also been isolated from other fungal genera like Penicillium. [, ]
Q2: What are the known biological activities of this compound?
A2: this compound exhibits a range of biological activities, most notably as an antioxidant. It demonstrates strong 1,1-diphenyl-2-picrylhydrazyl (DPPH) radical scavenging activity, comparable to the known antioxidant ferulic acid. [, , ] This antioxidant capacity suggests potential benefits against oxidative stress. Additionally, some studies suggest potential anti-tumor promoting activity. []
Q3: Does the International Space Station environment impact this compound production?
A3: Interestingly, research has shown that Aspergillus niger JSC-093350089, when grown on the International Space Station, exhibited altered molecular responses compared to its ground-grown counterpart. [] This included significantly enhanced production of this compound after the strain was returned to Earth and re-grown, suggesting a potential role for this compound in the fungal response to the unique stressors of space. []
Q4: How is this compound biosynthesized?
A4: Research indicates that this compound biosynthesis involves a complex pathway utilizing a polyketide synthase (PKS) and nonribosomal peptide synthetase (NRPS) hybrid enzyme. [] This pathway utilizes four units of acetate and one unit of glycine to form the compound's core structure. [] Further research revealed the involvement of several key enzymes, including two redox enzymes crucial for forming the fused γ-pyrone core and a protease homologue responsible for exo-methylene formation. []
Q5: Are there specific genes involved in this compound biosynthesis?
A5: Yes, a biosynthetic gene cluster responsible for this compound production has been identified in Aspergillus niger. [, ] This cluster includes a previously uncharacterized gene, pyrE, playing a role in the biosynthesis. [] Further research identified the pathway-specific transcriptional regulator PynR, which, when overexpressed, activates the this compound biosynthetic gene cluster. []
Q6: How is this compound production regulated in Aspergillus niger?
A6: Studies in Aspergillus niger show that the sporulation gene flbA, which encodes a Regulator of G-protein Signaling, indirectly influences this compound production. [] Deletion of flbA impacts the expression of various transcription factors, including Fum21. [] Fum21, activated by FlbA, plays a significant role in regulating the expression of genes within the fumonisin biosynthetic cluster, which is closely linked to this compound production. [] Deletion of fum21 significantly reduces this compound production, highlighting the interconnected regulatory network governing secondary metabolite production in this fungus. []
Disclaimer and Information on In-Vitro Research Products
Please be aware that all articles and product information presented on BenchChem are intended solely for informational purposes. The products available for purchase on BenchChem are specifically designed for in-vitro studies, which are conducted outside of living organisms. In-vitro studies, derived from the Latin term "in glass," involve experiments performed in controlled laboratory settings using cells or tissues. It is important to note that these products are not categorized as medicines or drugs, and they have not received approval from the FDA for the prevention, treatment, or cure of any medical condition, ailment, or disease. We must emphasize that any form of bodily introduction of these products into humans or animals is strictly prohibited by law. It is essential to adhere to these guidelines to ensure compliance with legal and ethical standards in research and experimentation.