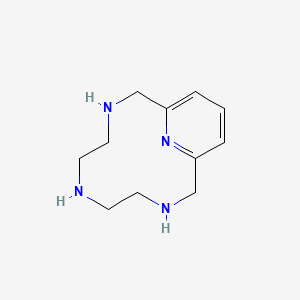
1,4,7,10-Tetraaza-2,6-pyridinophane
Overview
Description
1,4,7,10-Tetraaza-2,6-pyridinophane is a macrocyclic compound known for its ability to form stable complexes with metal ions. This compound is particularly significant in the field of diagnostic imaging, where it is used as a ligand for essential metal ion-based MRI and PET contrast agents .
Mechanism of Action
Target of Action
Pyclen, also known as 3,6,9,15-Tetraazabicyclo[9.3.1]pentadeca-1(15),11,13-triene, is primarily used as a contrast agent in magnetic resonance imaging (MRI) and is known to target lesions with abnormal vascularity in the central nervous system and the body . It is a gadolinium-based contrast agent (GBCA) based on a pyclen macrocyclic structure .
Mode of Action
Pyclen is a macrocyclic non-ionic complex of gadolinium and a paramagnetic molecule that develops a magnetic moment when placed in a magnetic field . The gadolinium ion in the Pyclen complex interacts with water molecules in the body, altering the relaxation rates of the water protons and enhancing the contrast in the MRI images .
Pharmacokinetics
It is known that pyclen has high kinetic stability, allowing it to be used at lower doses than classic extracellular gbcas
Preparation Methods
Synthetic Routes and Reaction Conditions
The synthesis of 1,4,7,10-Tetraaza-2,6-pyridinophane typically involves the reaction of tert-butyl bromoacetate with the compound in dry acetonitrile. The reaction is facilitated by the presence of potassium carbonate as a base . The commercially available tert-butyl bromoacetate (0.27 g, 1.40 mmol, 0.20 ml, 2.2 equivalent) is dissolved in dry acetonitrile and added dropwise to the acetonitrile solution (30 ml) of 3,6,9,15-Tetraazabicyclo[9.3.1]pentadeca-1(14),11(15),12-triene-13-methoxy (0.15 g, 0.63 mmol, 1 equivalent) and potassium carbonate (0.26 g, 1.89 mmol, 3 equivalent) .
Industrial Production Methods
Industrial production methods for this compound are not widely documented. the synthesis process can be scaled up by maintaining the reaction conditions and using industrial-grade reagents and solvents.
Chemical Reactions Analysis
Types of Reactions
1,4,7,10-Tetraaza-2,6-pyridinophane undergoes various chemical reactions, including:
Complexation: Forms stable complexes with metal ions such as Mn(II), Fe(II), Fe(III), Co(II), and Ni(II).
Substitution: Reacts with tert-butyl bromoacetate in the presence of potassium carbonate.
Common Reagents and Conditions
Reagents: Tert-butyl bromoacetate, potassium carbonate, dry acetonitrile.
Conditions: Reactions are typically carried out in dry acetonitrile at room temperature.
Major Products
Scientific Research Applications
1,4,7,10-Tetraaza-2,6-pyridinophane has several scientific research applications:
Diagnostic Imaging: Used as a ligand for essential metal ion-based MRI and PET contrast agents.
Therapeutic Applications: Potential use in radical scavenging and metal chelation.
Nanoparticle Engineering: Immobilized onto dense silica nanoparticles for various applications.
Comparison with Similar Compounds
Similar Compounds
1,4,7,10-Tetraazacyclododecane-1,4,7,10-tetraacetic acid (DOTA): Another macrocyclic compound used as a chelating agent for metal ions in diagnostic imaging.
1,4,7,10-Tetraaza-2,6-pyridinophane-3,6,9-triacetic acid (PCTA): A derivative of the compound with additional carboxylic acid groups.
Uniqueness
This compound is unique due to its specific structure that allows for the formation of highly stable metal complexes. This stability is crucial for its effectiveness as a contrast agent in diagnostic imaging .
Properties
IUPAC Name |
3,6,9,15-tetrazabicyclo[9.3.1]pentadeca-1(15),11,13-triene | |
---|---|---|
Source | PubChem | |
URL | https://pubchem.ncbi.nlm.nih.gov | |
Description | Data deposited in or computed by PubChem | |
InChI |
InChI=1S/C11H18N4/c1-2-10-8-13-6-4-12-5-7-14-9-11(3-1)15-10/h1-3,12-14H,4-9H2 | |
Source | PubChem | |
URL | https://pubchem.ncbi.nlm.nih.gov | |
Description | Data deposited in or computed by PubChem | |
InChI Key |
LNMUPMQUMCDOKO-UHFFFAOYSA-N | |
Source | PubChem | |
URL | https://pubchem.ncbi.nlm.nih.gov | |
Description | Data deposited in or computed by PubChem | |
Canonical SMILES |
C1CNCC2=NC(=CC=C2)CNCCN1 | |
Source | PubChem | |
URL | https://pubchem.ncbi.nlm.nih.gov | |
Description | Data deposited in or computed by PubChem | |
Molecular Formula |
C11H18N4 | |
Source | PubChem | |
URL | https://pubchem.ncbi.nlm.nih.gov | |
Description | Data deposited in or computed by PubChem | |
DSSTOX Substance ID |
DTXSID10343011 | |
Record name | 3,6,9,15-Tetraazabicyclo[9.3.1]pentadeca-1(15),11,13-triene | |
Source | EPA DSSTox | |
URL | https://comptox.epa.gov/dashboard/DTXSID10343011 | |
Description | DSSTox provides a high quality public chemistry resource for supporting improved predictive toxicology. | |
Molecular Weight |
206.29 g/mol | |
Source | PubChem | |
URL | https://pubchem.ncbi.nlm.nih.gov | |
Description | Data deposited in or computed by PubChem | |
CAS No. |
78668-34-5 | |
Record name | 3,6,9,15-Tetraazabicyclo[9.3.1]pentadeca-1(15),11,13-triene | |
Source | EPA DSSTox | |
URL | https://comptox.epa.gov/dashboard/DTXSID10343011 | |
Description | DSSTox provides a high quality public chemistry resource for supporting improved predictive toxicology. | |
Synthesis routes and methods
Procedure details
Retrosynthesis Analysis
AI-Powered Synthesis Planning: Our tool employs the Template_relevance Pistachio, Template_relevance Bkms_metabolic, Template_relevance Pistachio_ringbreaker, Template_relevance Reaxys, Template_relevance Reaxys_biocatalysis model, leveraging a vast database of chemical reactions to predict feasible synthetic routes.
One-Step Synthesis Focus: Specifically designed for one-step synthesis, it provides concise and direct routes for your target compounds, streamlining the synthesis process.
Accurate Predictions: Utilizing the extensive PISTACHIO, BKMS_METABOLIC, PISTACHIO_RINGBREAKER, REAXYS, REAXYS_BIOCATALYSIS database, our tool offers high-accuracy predictions, reflecting the latest in chemical research and data.
Strategy Settings
Precursor scoring | Relevance Heuristic |
---|---|
Min. plausibility | 0.01 |
Model | Template_relevance |
Template Set | Pistachio/Bkms_metabolic/Pistachio_ringbreaker/Reaxys/Reaxys_biocatalysis |
Top-N result to add to graph | 6 |
Feasible Synthetic Routes
Q1: What is the molecular formula and weight of Pyclen?
A1: The molecular formula of Pyclen is C11H18N4, and its molecular weight is 206.29 g/mol.
Q2: What spectroscopic data is available for Pyclen and its complexes?
A2: Various spectroscopic techniques are employed to characterize Pyclen and its metal complexes. These include:
- NMR Spectroscopy (1H, 13C, 89Y, 31P): Used to determine protonation sequences, study complex structures in solution, analyze metal-induced shifts, and investigate dynamics. [, , , , , , , , , , , , , , , , , , , , , , , , , , , ]
- UV-Vis Spectroscopy: Employed to investigate complex formation, determine stability constants, and study redox properties. [, , , , , , , , , , , , , , , , , , , , , , , , , , , ]
- Luminescence Spectroscopy: Used to study the photophysical properties of lanthanide complexes, including emission spectra, quantum yields, and lifetimes. [, , , , , , , ]
- EPR Spectroscopy: Employed to investigate the electronic structure and geometry of paramagnetic metal complexes, particularly Cu(II) complexes. [, ]
- X-ray Diffraction: Used to determine the solid-state structures of Pyclen, its derivatives, and their metal complexes. [, , , , , , , , , , , , , , , , , , , , , , , , , , ]
Q3: Is Pyclen stable under various conditions?
A3: Pyclen itself is a stable compound. The stability of its metal complexes is influenced by factors such as:
- pH: Stability generally decreases with decreasing pH due to proton-assisted dissociation. [, , , , , , , , , , , , , , , , , , , , , , , , , , ]
- Presence of Competing Metal Ions: The presence of metal ions with high affinity for the ligand can lead to transmetalation. [, ]
- Ligand Modification: Structural modifications of the Pyclen scaffold can significantly impact the kinetic inertness and thermodynamic stability of the resulting metal complexes. [, , , , , , , , , , , , , , , , , , , , , , , , , , ]
Q4: What are the applications of Pyclen and its derivatives under various conditions?
A4: Pyclen and its derivatives have been explored for diverse applications due to their ability to form stable complexes with various metal ions. These applications include:
- Magnetic Resonance Imaging (MRI) Contrast Agents: Gd(III) complexes of Pyclen derivatives have shown promise as MRI contrast agents, particularly for liver-specific imaging. [, , , , , , ]
- Radiopharmaceuticals: Pyclen-based chelators have been investigated for radiolabeling with β−-emitters like 90Y for potential use in β-radiotherapy, particularly for liver and brain cancers. [, , ]
- Luminescent Bioprobes: Lanthanide complexes of Pyclen derivatives, especially those containing picolinate antennas, exhibit interesting luminescent properties, making them suitable for in vitro and in vivo bioimaging applications, including two-photon microscopy. [, , ]
- Catalysts: Metal complexes of Pyclen derivatives have shown catalytic activity in reactions such as hydrogen peroxide disproportionation, mimicking the activity of catalase enzymes. []
- Superoxide Dismutase (SOD) Mimics: Cu(II) complexes of Pyclen derivatives have been studied as SOD mimics, exhibiting the potential for therapeutic applications related to oxidative stress. []
Q5: How is computational chemistry employed in Pyclen research?
A5: Computational methods, such as Density Functional Theory (DFT), are extensively used in Pyclen research to:
- Predict Molecular Geometries: DFT calculations help determine the most stable conformations of Pyclen derivatives and their metal complexes in solution and solid state. [, , , , , , , ]
- Rationalize Experimental Observations: Computational studies provide insights into the electronic structure, bonding interactions, and spectroscopic properties of Pyclen complexes, aiding in the interpretation of experimental data. [, , , ]
- Guide Ligand Design: DFT calculations are valuable tools for predicting the properties of new Pyclen derivatives and their metal complexes, guiding the synthesis of compounds with desired characteristics. [, , ]
Q6: How do structural modifications of Pyclen affect its activity and properties?
A6: The Structure-Activity Relationship (SAR) of Pyclen derivatives is heavily influenced by modifications to its scaffold. Key structural changes and their effects include:
- Pendant Arms: The nature, number, and position of pendant arms significantly influence the stability, kinetic inertness, and relaxivity of metal complexes. For example, picolinate arms enhance thermodynamic stability and luminescence properties, while acetate arms can be used to tune water exchange rates and relaxivity. [, , , , , , , , , , , , , , , , , , , , , , , , , , ]
- Pyridine Functionalization: Modifications to the pyridine ring, such as hydroxylation or substitution with electron-withdrawing or -donating groups, can impact the electronic properties of the ligand, affecting the redox potential, stability, and catalytic activity of the resulting metal complexes. [, , ]
- Capping Bond Effect: The nature of the bonds formed between the metal ion and the donor atoms of the ligand, particularly around the metal coordination environment, significantly influences the stability and kinetic inertness of the complex. This effect is particularly pronounced in dissymmetrically functionalized Pyclen derivatives. []
Q7: What strategies are employed to improve the stability and bioavailability of Pyclen-based compounds?
A7: Various strategies are employed to enhance the stability and bioavailability of Pyclen-based compounds:
- Lipophilic Modifications: Incorporating lipophilic groups, such as alkyl chains, can improve the lipophilicity of the complexes, potentially enhancing their cellular uptake and bioavailability. [, ]
- Conjugation to Targeting Vectors: Conjugating Pyclen-based chelates to biomolecules, such as peptides or antibodies, can improve their targeting ability and delivery to specific tissues or cells. [, , ]
Q8: What analytical methods are used to characterize and quantify Pyclen and its derivatives?
A8: A combination of analytical techniques is employed to characterize and quantify Pyclen and its derivatives:
- Potentiometric Titration: Used to determine protonation constants of the ligand and stability constants of metal complexes. [, , , , , , , , , , , , , , , , , , , , , , , , , , ]
- Electrospray Ionization Mass Spectrometry (ESI-MS): Provides information on the molecular weight and fragmentation patterns of the compounds. []
Q9: What is known about the toxicity and safety of Pyclen and its derivatives?
A9: While Pyclen itself is considered relatively non-toxic, the toxicity of its metal complexes depends on various factors, including the metal ion, the ligand structure, the dose, and the route of administration.
- In Vitro Toxicity: Some Pyclen-Gd(III) complexes have shown minimal toxicity in cell-based assays. []
- Gd Toxicity: Concerns regarding the toxicity of Gd(III)-based contrast agents, particularly in patients with kidney failure, have fueled the search for alternative metal ions like Mn(II). [, , , ]
Q10: What are the environmental implications of Pyclen and its derivatives?
A10: Limited information is available on the environmental impact and degradation of Pyclen and its derivatives.
Q11: What are the future directions for research on Pyclen and its derivatives?
A11: Ongoing research on Pyclen and its derivatives continues to expand its applications in various fields:
- Development of Safer and More Effective MRI Contrast Agents: Research focuses on developing Mn(II)-based complexes with improved relaxivity and pharmacokinetic profiles while minimizing potential toxicity. [, , , ]
- Targeted Radionuclide Therapy: Efforts are underway to develop Pyclen-based chelators for radiolabeling with therapeutic radionuclides for targeted cancer therapy. [, , ]
- Design of Multimodal Imaging Probes: Combining Pyclen-based chelates with different imaging modalities, such as MRI and optical imaging, holds promise for improved disease diagnosis and monitoring. []
- Exploration of Catalytic Applications: Investigating the catalytic potential of Pyclen-metal complexes, particularly in reactions relevant to biological systems or environmental remediation, remains an active area of research. [, ]
Disclaimer and Information on In-Vitro Research Products
Please be aware that all articles and product information presented on BenchChem are intended solely for informational purposes. The products available for purchase on BenchChem are specifically designed for in-vitro studies, which are conducted outside of living organisms. In-vitro studies, derived from the Latin term "in glass," involve experiments performed in controlled laboratory settings using cells or tissues. It is important to note that these products are not categorized as medicines or drugs, and they have not received approval from the FDA for the prevention, treatment, or cure of any medical condition, ailment, or disease. We must emphasize that any form of bodily introduction of these products into humans or animals is strictly prohibited by law. It is essential to adhere to these guidelines to ensure compliance with legal and ethical standards in research and experimentation.