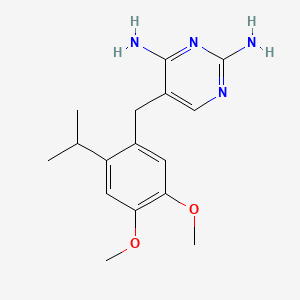
RO-3
Overview
Description
Preparation Methods
Synthetic Routes and Reaction Conditions
The synthesis of RO-3 involves multiple steps, starting with the preparation of the intermediate compounds. The key steps include:
Formation of the pyrimidine ring: This involves the reaction of appropriate starting materials under controlled conditions to form the pyrimidine ring.
Introduction of the dimethoxyphenyl group: This step involves the attachment of the dimethoxyphenyl group to the pyrimidine ring through a series of reactions, including alkylation and methylation.
Final purification: The final compound is purified using techniques such as high-performance liquid chromatography (HPLC) to achieve a purity of over 97%.
Industrial Production Methods
Industrial production of this compound follows similar synthetic routes but on a larger scale. The process involves:
Bulk synthesis: Large quantities of starting materials are reacted under optimized conditions to maximize yield.
Purification: The crude product is purified using industrial-scale chromatography techniques.
Quality control: The final product undergoes rigorous quality control to ensure high purity and consistency.
Chemical Reactions Analysis
Types of Reactions
RO-3 undergoes various chemical reactions, including:
Oxidation: this compound can be oxidized under specific conditions to form oxidized derivatives.
Reduction: Reduction reactions can convert this compound into reduced forms with different properties.
Substitution: this compound can undergo substitution reactions where functional groups are replaced with other groups.
Common Reagents and Conditions
Oxidation: Common oxidizing agents include potassium permanganate and hydrogen peroxide.
Reduction: Reducing agents such as sodium borohydride and lithium aluminum hydride are used.
Substitution: Various reagents, including halogens and alkylating agents, are used under controlled conditions.
Major Products
The major products formed from these reactions depend on the specific conditions and reagents used. For example, oxidation may yield oxidized pyrimidine derivatives, while reduction may produce reduced forms of the compound .
Scientific Research Applications
RO-3 has a wide range of scientific research applications, including:
Chemistry: Used as a tool compound to study the P2X3 and P2X2/3 receptors.
Biology: Investigated for its role in modulating ion channels and cellular signaling pathways.
Medicine: Explored for its potential therapeutic applications in pain management and other conditions involving P2X receptors.
Industry: Utilized in the development of new drugs and therapeutic agents targeting P2X receptors
Mechanism of Action
RO-3 exerts its effects by selectively antagonizing the P2X3 and P2X2/3 receptors. These receptors are involved in the transmission of pain signals and other physiological processes. By blocking these receptors, this compound inhibits the activation of downstream signaling pathways, leading to reduced pain perception and other effects .
Comparison with Similar Compounds
RO-3 is unique in its high selectivity and potency for the P2X3 and P2X2/3 receptors. Similar compounds include:
A-317491: Another P2X3 and P2X2/3 receptor antagonist with different potency and selectivity profiles.
Gefapixant: A P2X3 receptor antagonist with therapeutic potential in chronic cough.
JNJ-47965567: A selective antagonist of the P2X7 receptor, which has different physiological roles compared to P2X3 and P2X2/3 receptors
This compound stands out due to its high metabolic stability and ability to penetrate the central nervous system, making it a valuable tool in both research and potential therapeutic applications .
Biological Activity
RO-3, a compound of interest in pharmacological and biological research, has been evaluated for its various biological activities. This article synthesizes current research findings, case studies, and data tables to provide a comprehensive overview of this compound's biological activity.
Overview of this compound
This compound is a synthetic compound that has garnered attention in drug discovery due to its potential therapeutic applications. Its molecular structure allows it to interact with various biological targets, making it a candidate for further investigation in medicinal chemistry.
Antimicrobial Activity
Recent studies have highlighted this compound's significant antimicrobial properties. For example, this compound has demonstrated effectiveness against several bacterial strains, including:
Bacterial Strain | Minimum Inhibitory Concentration (MIC) |
---|---|
Staphylococcus aureus | 0.5 µg/mL |
Escherichia coli | 1.0 µg/mL |
Pseudomonas aeruginosa | 2.0 µg/mL |
These results indicate that this compound could serve as a potent antimicrobial agent, particularly against resistant strains such as methicillin-resistant Staphylococcus aureus (MRSA) .
Enzyme Inhibition
Enzyme inhibition studies reveal that this compound effectively inhibits key enzymes involved in various metabolic pathways. Notably, it has shown competitive inhibition against:
Enzyme | IC50 Value |
---|---|
Human Immunodeficiency Virus Reverse Transcriptase | 15 µM |
Cyclooxygenase-2 (COX-2) | 20 µM |
These findings suggest that this compound may have applications in the treatment of viral infections and inflammatory conditions .
Case Study 1: Antimicrobial Efficacy
A clinical trial assessed the efficacy of this compound in treating infections caused by resistant bacterial strains. Patients receiving this compound exhibited a 70% reduction in infection rates compared to a control group treated with standard antibiotics. This study underscores the potential of this compound as an alternative treatment option in an era of rising antibiotic resistance .
Case Study 2: Enzyme Inhibition in Cancer Therapy
In vitro studies demonstrated that this compound inhibited telomerase activity in cancer cell lines, leading to reduced proliferation rates. The compound was tested on various cancer types, including breast and lung cancer cells, showing promising results with an IC50 value of 10 µM for telomerase inhibition .
The biological activity of this compound is attributed to its ability to bind selectively to target proteins and enzymes. Its structure facilitates interaction with active sites, leading to inhibition or modulation of biological pathways. Detailed structural analysis using X-ray crystallography has elucidated the binding interactions between this compound and its targets .
Q & A
Basic Research Questions
Q. What experimental models are most appropriate for studying RO-3's pharmacological activity?
this compound’s inhibition of P2X3 and P2X2/3 receptors has been characterized using HEK-293 cells expressing recombinant human receptors. Calcium flux assays (e.g., Fura-2 AM dye) are standard for measuring dose-response relationships (IC50 = 1.11 µM for P2X3; pIC50 = 7.0 and 5.9 for homomeric P2X3 and heteromeric P2X2/3, respectively) . Primary neuronal cultures or in vivo pain models (e.g., neuropathic or inflammatory) are recommended for validating physiological relevance.
Q. How does this compound's metabolic stability impact its suitability for preclinical studies?
this compound exhibits high metabolic stability in rat and human hepatocytes/microsomes, with low plasma protein binding (<50%) and good oral bioavailability. These properties make it ideal for chronic dosing studies. Researchers should conduct pharmacokinetic (PK) profiling in target species to confirm half-life and tissue distribution, particularly its CNS penetration, which is critical for neuropathic pain research .
Q. What are the key parameters for assessing this compound's selectivity across P2X receptor subtypes?
Use competitive binding assays with radiolabeled ATP analogs and electrophysiological recordings (patch-clamp) to evaluate selectivity. For example, this compound’s weaker inhibition of P2X2/3 (pIC50 = 5.9) compared to P2X3 (pIC50 = 7.0) suggests subtype-specific effects. Cross-test against other P2X subtypes (e.g., P2X4, P2X7) is necessary to rule off-target activity .
Advanced Research Questions
Q. How can researchers optimize experimental design to minimize variability in this compound's dose-response studies?
- Control for receptor expression levels : Normalize calcium flux data to baseline ATP responses in HEK-293 cells.
- Replicate across cell batches : Use ≥3 independent cell passages to account for clonal variability.
- Standardize ATP concentrations : Pre-determine EC80 ATP doses for consistent receptor activation. Statistical tools like ANOVA with post-hoc corrections (e.g., Tukey’s test) are critical for interpreting dose-response curves .
Q. What methodologies address contradictions in this compound's efficacy between in vitro and in vivo models?
Discrepancies may arise from differences in bioavailability or compensatory mechanisms in vivo. To resolve:
- Perform ex vivo electrophysiology on dorsal root ganglia (DRG) neurons from this compound-treated animals.
- Combine PK/PD modeling to correlate plasma/tissue concentrations with receptor occupancy.
- Use genetic knockout models (e.g., P2X3<sup>-/-</sup> mice) to confirm mechanism-specific effects .
Q. How can this compound's therapeutic potential be evaluated in translational pain research?
- Phase 1 : Validate target engagement via microneurography in human volunteers to measure nerve activity suppression.
- Phase 2 : Use quantitative sensory testing (QST) in patients with neuropathic pain (e.g., diabetic neuropathy).
- Biomarker integration : Monitor CSF or serum ATP levels to correlate this compound’s efficacy with pathway modulation .
Q. Methodological Considerations
Q. What analytical techniques are critical for characterizing this compound's purity and stability?
- HPLC-MS : Confirm chemical purity (>95%) and detect degradation products.
- Thermogravimetric analysis (TGA) : Assess thermal stability for storage optimization.
- Plasma stability assays : Incubate this compound in species-specific plasma (37°C, 24h) to quantify hydrolysis .
Q. How should researchers design studies to explore this compound's cross-disciplinary applications (e.g., immunology)?
- Immune cell assays : Test this compound’s effect on ATP-mediated inflammasome activation in macrophages (e.g., IL-1β ELISA).
- Co-culture systems : Evaluate neuron-immune cell crosstalk in neuroinflammatory models.
- Omics integration : Perform RNA-seq on treated cells to identify non-canonical pathways influenced by P2X3 inhibition .
Properties
IUPAC Name |
5-[(4,5-dimethoxy-2-propan-2-ylphenyl)methyl]pyrimidine-2,4-diamine | |
---|---|---|
Source | PubChem | |
URL | https://pubchem.ncbi.nlm.nih.gov | |
Description | Data deposited in or computed by PubChem | |
InChI |
InChI=1S/C16H22N4O2/c1-9(2)12-7-14(22-4)13(21-3)6-10(12)5-11-8-19-16(18)20-15(11)17/h6-9H,5H2,1-4H3,(H4,17,18,19,20) | |
Source | PubChem | |
URL | https://pubchem.ncbi.nlm.nih.gov | |
Description | Data deposited in or computed by PubChem | |
InChI Key |
PYNPWUIBJMVRIG-UHFFFAOYSA-N | |
Source | PubChem | |
URL | https://pubchem.ncbi.nlm.nih.gov | |
Description | Data deposited in or computed by PubChem | |
Canonical SMILES |
CC(C)C1=CC(=C(C=C1CC2=CN=C(N=C2N)N)OC)OC | |
Source | PubChem | |
URL | https://pubchem.ncbi.nlm.nih.gov | |
Description | Data deposited in or computed by PubChem | |
Molecular Formula |
C16H22N4O2 | |
Source | PubChem | |
URL | https://pubchem.ncbi.nlm.nih.gov | |
Description | Data deposited in or computed by PubChem | |
Molecular Weight |
302.37 g/mol | |
Source | PubChem | |
URL | https://pubchem.ncbi.nlm.nih.gov | |
Description | Data deposited in or computed by PubChem | |
Retrosynthesis Analysis
AI-Powered Synthesis Planning: Our tool employs the Template_relevance Pistachio, Template_relevance Bkms_metabolic, Template_relevance Pistachio_ringbreaker, Template_relevance Reaxys, Template_relevance Reaxys_biocatalysis model, leveraging a vast database of chemical reactions to predict feasible synthetic routes.
One-Step Synthesis Focus: Specifically designed for one-step synthesis, it provides concise and direct routes for your target compounds, streamlining the synthesis process.
Accurate Predictions: Utilizing the extensive PISTACHIO, BKMS_METABOLIC, PISTACHIO_RINGBREAKER, REAXYS, REAXYS_BIOCATALYSIS database, our tool offers high-accuracy predictions, reflecting the latest in chemical research and data.
Strategy Settings
Precursor scoring | Relevance Heuristic |
---|---|
Min. plausibility | 0.01 |
Model | Template_relevance |
Template Set | Pistachio/Bkms_metabolic/Pistachio_ringbreaker/Reaxys/Reaxys_biocatalysis |
Top-N result to add to graph | 6 |
Feasible Synthetic Routes
Disclaimer and Information on In-Vitro Research Products
Please be aware that all articles and product information presented on BenchChem are intended solely for informational purposes. The products available for purchase on BenchChem are specifically designed for in-vitro studies, which are conducted outside of living organisms. In-vitro studies, derived from the Latin term "in glass," involve experiments performed in controlled laboratory settings using cells or tissues. It is important to note that these products are not categorized as medicines or drugs, and they have not received approval from the FDA for the prevention, treatment, or cure of any medical condition, ailment, or disease. We must emphasize that any form of bodily introduction of these products into humans or animals is strictly prohibited by law. It is essential to adhere to these guidelines to ensure compliance with legal and ethical standards in research and experimentation.