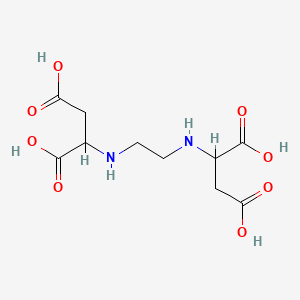
Trisodium ethylenediamine disuccinate
Overview
Description
Trisodium ethylenediamine disuccinate is a water-soluble compound widely used in various industries, particularly in cosmetics and personal care products. It functions primarily as a chelating agent, binding to metal ions such as calcium and iron to prevent them from causing undesirable reactions that could affect product stability and efficacy . This compound is derived from ethylenediamine, sodium hydroxide, and succinic acid .
Mechanism of Action
Target of Action
Ethylenediamine-N,N’-disuccinic acid (EDDS) is primarily a chelating agent . Its main targets are metal ions , with which it forms stable, water-soluble complexes .
Mode of Action
EDDS interacts with metal ions by forming stable water-soluble complexes . This interaction is due to the compound’s structure as an aminopolycarboxylic acid (APCA), which allows it to bind metal ions and form these complexes
Biochemical Analysis
Biochemical Properties
Trisodium ethylenediamine disuccinate plays a crucial role in biochemical reactions by forming stable complexes with metal ions. This property is particularly important in bioremediation, where it helps mobilize and remove heavy metals from contaminated environments. The compound interacts with various enzymes and proteins, including metal-dependent enzymes, by binding to metal ions and preventing their participation in catalytic processes. For example, this compound can inhibit the activity of metalloenzymes by chelating the metal cofactors required for their function .
Cellular Effects
This compound influences cellular processes by altering the availability of metal ions within cells. It can affect cell signaling pathways, gene expression, and cellular metabolism by chelating essential metal ions such as zinc, copper, and iron. This chelation can lead to changes in the activity of metal-dependent enzymes and transcription factors, ultimately impacting cellular functions. For instance, this compound has been shown to increase the bioavailability of heavy metals in plants, enhancing their uptake and accumulation .
Molecular Mechanism
The molecular mechanism of this compound involves its ability to form stable complexes with metal ions through its aminocarboxylic acid groups. This chelation process can inhibit or activate enzymes by altering the availability of metal cofactors. For example, this compound can inhibit the activity of metalloenzymes by binding to their metal cofactors, preventing them from participating in catalytic reactions. Additionally, the compound can influence gene expression by modulating the activity of metal-dependent transcription factors .
Temporal Effects in Laboratory Settings
In laboratory settings, the effects of this compound can change over time due to its stability and degradation. The compound is known for its biodegradability, with a degradation period ranging from 7 to 30 days . Over time, the chelation of metal ions by this compound can lead to changes in cellular function, including alterations in enzyme activity and gene expression. Long-term studies have shown that the compound can enhance the uptake of heavy metals in plants, leading to increased accumulation in tissues .
Dosage Effects in Animal Models
The effects of this compound vary with different dosages in animal models. At low doses, the compound can effectively chelate metal ions without causing significant toxicity. At high doses, this compound may exhibit toxic effects, including disruption of metal homeostasis and inhibition of essential metalloenzymes. Studies have shown that the compound can cause adverse effects on cellular function and overall health at high concentrations .
Metabolic Pathways
This compound is involved in various metabolic pathways, particularly those related to metal ion homeostasis. The compound interacts with enzymes and cofactors involved in metal transport and metabolism, influencing metabolic flux and metabolite levels. For example, this compound can affect the activity of enzymes involved in the synthesis and degradation of metal-containing compounds, leading to changes in cellular metabolism .
Transport and Distribution
Within cells and tissues, this compound is transported and distributed through interactions with transporters and binding proteins. The compound can be taken up by cells through specific transport mechanisms and subsequently distributed to various cellular compartments. This distribution is influenced by the compound’s ability to form stable complexes with metal ions, which can affect its localization and accumulation within cells .
Subcellular Localization
The subcellular localization of this compound is determined by its interactions with metal ions and cellular components. The compound can be localized to specific compartments or organelles, such as the cytoplasm, vacuoles, and cell walls, depending on its binding interactions. For example, in plant cells, this compound has been observed to accumulate in cell walls and vacuoles, where it forms complexes with metal ions .
Preparation Methods
Synthetic Routes and Reaction Conditions
Trisodium ethylenediamine disuccinate is synthesized through the condensation of ethylenediamine and succinic acid, followed by the addition of sodium hydroxide to form the trisodium salt . The reaction typically involves:
Condensation: Ethylenediamine reacts with succinic acid under controlled conditions.
Neutralization: Sodium hydroxide is added to neutralize the reaction mixture, forming the trisodium salt.
Purification: The resulting compound is purified to obtain the final product.
Industrial Production Methods
In industrial settings, the production of this compound follows similar steps but on a larger scale. The process involves:
Bulk Reactors: Large reactors are used to facilitate the condensation and neutralization reactions.
Continuous Processing: The reactions are often carried out in a continuous process to enhance efficiency and yield.
Purification and Quality Control: The final product undergoes rigorous purification and quality control to ensure consistency and purity.
Chemical Reactions Analysis
Types of Reactions
Trisodium ethylenediamine disuccinate primarily undergoes chelation reactions, where it binds to metal ions. It does not typically participate in oxidation, reduction, or substitution reactions under standard conditions .
Common Reagents and Conditions
Chelation: The compound readily forms stable complexes with metal ions such as calcium, iron, and magnesium.
Conditions: These reactions generally occur in aqueous solutions at neutral to slightly alkaline pH levels.
Major Products Formed
The major products formed from the chelation reactions of this compound are metal complexes, which are stable and water-soluble .
Scientific Research Applications
Trisodium ethylenediamine disuccinate has a wide range of applications in scientific research, including:
Comparison with Similar Compounds
Similar Compounds
Ethylenediaminetetraacetic acid (EDTA): Another widely used chelating agent with similar properties but less biodegradable.
Tetrasodium iminodisuccinate: A structurally similar compound used in similar applications.
Uniqueness
Trisodium ethylenediamine disuccinate stands out due to its higher biodegradability compared to other chelating agents like EDTA . This makes it a more environmentally friendly option for various applications, particularly in industries focused on sustainability .
Properties
IUPAC Name |
(2S)-2-[2-[[(1S)-1,2-dicarboxyethyl]amino]ethylamino]butanedioic acid | |
---|---|---|
Source | PubChem | |
URL | https://pubchem.ncbi.nlm.nih.gov | |
Description | Data deposited in or computed by PubChem | |
InChI |
InChI=1S/C10H16N2O8/c13-7(14)3-5(9(17)18)11-1-2-12-6(10(19)20)4-8(15)16/h5-6,11-12H,1-4H2,(H,13,14)(H,15,16)(H,17,18)(H,19,20)/t5-,6-/m0/s1 | |
Source | PubChem | |
URL | https://pubchem.ncbi.nlm.nih.gov | |
Description | Data deposited in or computed by PubChem | |
InChI Key |
VKZRWSNIWNFCIQ-WDSKDSINSA-N | |
Source | PubChem | |
URL | https://pubchem.ncbi.nlm.nih.gov | |
Description | Data deposited in or computed by PubChem | |
Canonical SMILES |
C(CNC(CC(=O)O)C(=O)O)NC(CC(=O)O)C(=O)O | |
Source | PubChem | |
URL | https://pubchem.ncbi.nlm.nih.gov | |
Description | Data deposited in or computed by PubChem | |
Isomeric SMILES |
C(CN[C@@H](CC(=O)O)C(=O)O)N[C@@H](CC(=O)O)C(=O)O | |
Source | PubChem | |
URL | https://pubchem.ncbi.nlm.nih.gov | |
Description | Data deposited in or computed by PubChem | |
Molecular Formula |
C10H16N2O8 | |
Source | PubChem | |
URL | https://pubchem.ncbi.nlm.nih.gov | |
Description | Data deposited in or computed by PubChem | |
DSSTOX Substance ID |
DTXSID1051852 | |
Record name | Ethylenediamine-N,N′-disuccinic acid | |
Source | EPA DSSTox | |
URL | https://comptox.epa.gov/dashboard/DTXSID1051852 | |
Description | DSSTox provides a high quality public chemistry resource for supporting improved predictive toxicology. | |
Molecular Weight |
292.24 g/mol | |
Source | PubChem | |
URL | https://pubchem.ncbi.nlm.nih.gov | |
Description | Data deposited in or computed by PubChem | |
CAS No. |
20846-91-7 | |
Record name | Ethylenediamine-N,N′-disuccinic acid | |
Source | CAS Common Chemistry | |
URL | https://commonchemistry.cas.org/detail?cas_rn=20846-91-7 | |
Description | CAS Common Chemistry is an open community resource for accessing chemical information. Nearly 500,000 chemical substances from CAS REGISTRY cover areas of community interest, including common and frequently regulated chemicals, and those relevant to high school and undergraduate chemistry classes. This chemical information, curated by our expert scientists, is provided in alignment with our mission as a division of the American Chemical Society. | |
Explanation | The data from CAS Common Chemistry is provided under a CC-BY-NC 4.0 license, unless otherwise stated. | |
Record name | N,N'-Ethylenediamine disuccinic acid | |
Source | ChemIDplus | |
URL | https://pubchem.ncbi.nlm.nih.gov/substance/?source=chemidplus&sourceid=0020846917 | |
Description | ChemIDplus is a free, web search system that provides access to the structure and nomenclature authority files used for the identification of chemical substances cited in National Library of Medicine (NLM) databases, including the TOXNET system. | |
Record name | L-Aspartic acid, N,N'-1,2-ethanediylbis- | |
Source | EPA Chemicals under the TSCA | |
URL | https://www.epa.gov/chemicals-under-tsca | |
Description | EPA Chemicals under the Toxic Substances Control Act (TSCA) collection contains information on chemicals and their regulations under TSCA, including non-confidential content from the TSCA Chemical Substance Inventory and Chemical Data Reporting. | |
Record name | Ethylenediamine-N,N′-disuccinic acid | |
Source | EPA DSSTox | |
URL | https://comptox.epa.gov/dashboard/DTXSID1051852 | |
Description | DSSTox provides a high quality public chemistry resource for supporting improved predictive toxicology. | |
Record name | Ethylenediamine Disuccinic Acid | |
Source | European Chemicals Agency (ECHA) | |
URL | https://echa.europa.eu/information-on-chemicals | |
Description | The European Chemicals Agency (ECHA) is an agency of the European Union which is the driving force among regulatory authorities in implementing the EU's groundbreaking chemicals legislation for the benefit of human health and the environment as well as for innovation and competitiveness. | |
Explanation | Use of the information, documents and data from the ECHA website is subject to the terms and conditions of this Legal Notice, and subject to other binding limitations provided for under applicable law, the information, documents and data made available on the ECHA website may be reproduced, distributed and/or used, totally or in part, for non-commercial purposes provided that ECHA is acknowledged as the source: "Source: European Chemicals Agency, http://echa.europa.eu/". Such acknowledgement must be included in each copy of the material. ECHA permits and encourages organisations and individuals to create links to the ECHA website under the following cumulative conditions: Links can only be made to webpages that provide a link to the Legal Notice page. | |
Record name | ETHYLENEDIAMINEDISUCCINIC ACID, (S,S)- | |
Source | FDA Global Substance Registration System (GSRS) | |
URL | https://gsrs.ncats.nih.gov/ginas/app/beta/substances/5WK2FGJ113 | |
Description | The FDA Global Substance Registration System (GSRS) enables the efficient and accurate exchange of information on what substances are in regulated products. Instead of relying on names, which vary across regulatory domains, countries, and regions, the GSRS knowledge base makes it possible for substances to be defined by standardized, scientific descriptions. | |
Explanation | Unless otherwise noted, the contents of the FDA website (www.fda.gov), both text and graphics, are not copyrighted. They are in the public domain and may be republished, reprinted and otherwise used freely by anyone without the need to obtain permission from FDA. Credit to the U.S. Food and Drug Administration as the source is appreciated but not required. | |
Synthesis routes and methods
Procedure details
Retrosynthesis Analysis
AI-Powered Synthesis Planning: Our tool employs the Template_relevance Pistachio, Template_relevance Bkms_metabolic, Template_relevance Pistachio_ringbreaker, Template_relevance Reaxys, Template_relevance Reaxys_biocatalysis model, leveraging a vast database of chemical reactions to predict feasible synthetic routes.
One-Step Synthesis Focus: Specifically designed for one-step synthesis, it provides concise and direct routes for your target compounds, streamlining the synthesis process.
Accurate Predictions: Utilizing the extensive PISTACHIO, BKMS_METABOLIC, PISTACHIO_RINGBREAKER, REAXYS, REAXYS_BIOCATALYSIS database, our tool offers high-accuracy predictions, reflecting the latest in chemical research and data.
Strategy Settings
Precursor scoring | Relevance Heuristic |
---|---|
Min. plausibility | 0.01 |
Model | Template_relevance |
Template Set | Pistachio/Bkms_metabolic/Pistachio_ringbreaker/Reaxys/Reaxys_biocatalysis |
Top-N result to add to graph | 6 |
Feasible Synthetic Routes
Q1: How does EDDS enhance the phytoextraction of heavy metals from contaminated soils?
A1: EDDS forms strong complexes with heavy metal ions in the soil, increasing their solubility and mobility. This enhanced mobility facilitates the uptake and translocation of heavy metals by plant roots, leading to higher accumulation in plant tissues, a process known as phytoextraction. [, , , , , , , ]
Q2: Does the effectiveness of EDDS in phytoextraction vary depending on the specific metal contaminant?
A2: Yes, the effectiveness of EDDS varies depending on the target metal. Research has shown that EDDS is particularly effective in enhancing the phytoextraction of copper (Cu) and zinc (Zn) but less effective for lead (Pb). [, , , ]
Q3: What are the implications of EDDS application on soil microbial activity?
A3: Research suggests that EDDS application, compared to traditional chelators like EDTA, has a less detrimental effect on soil microbial activity. Studies utilizing Biolog ECO MicroPlates have demonstrated that EDDS-treated soils exhibit higher substrate utilization by soil microorganisms, indicating a healthier microbial community. []
Q4: What is the molecular formula and weight of EDDS?
A4: The molecular formula of EDDS is C10H16N2O8. Its molecular weight is 292.24 g/mol.
Q5: Is there any spectroscopic data available for the characterization of EDDS and its metal complexes?
A5: Yes, Fourier Transform Infrared Spectroscopy (FTIR) has been used to characterize EDDS and its metal complexes. FTIR analysis reveals changes in the number and strength of functional groups in the solution, explaining the enhanced chelating ability of EDDS towards heavy metal ions. []
Q6: How does the performance of EDDS in removing organic pollutants compare to other methods like UV-H2O2?
A6: Studies indicate that the UV-Fenton process utilizing EDDS as a chelating agent exhibits higher efficiency in removing organic pollutants compared to UV-H2O2. For instance, the removal of Naphthenic acids (NAs) was significantly higher in the UV-EDDS-Fenton process than in UV-H2O2. []
Q7: Does the presence of organic matter or inorganic salts in water affect the effectiveness of EDDS in pollutant removal?
A7: Yes, the presence of organic matter and inorganic salts can influence the effectiveness of EDDS. Studies using EDDS in a photo-Fenton process for treating pineapple processing wastewater showed that components like nitrites and carbonates can significantly impact the pollutant removal efficiency. []
Q8: How does EDDS contribute to the activation of peroxymonosulfate (PMS) in electrochemical advanced oxidation processes?
A8: EDDS forms a stable complex with iron (Fe), enhancing the redox cycle of Fe(III)-EDDS/Fe(II)-EDDS. This enhanced redox cycle effectively activates PMS, generating reactive oxygen species (ROS) like •OH, 1O2, and SO4•−, which are responsible for the degradation of organic pollutants. []
Q9: Have computational methods been employed to study the complexation of EDDS with metal ions?
A9: Yes, Density Functional Theory (DFT) calculations have been employed to investigate the complexation of EDDS with various metal ions. These calculations provide insights into the stability, geometry, and electronic properties of the formed complexes. []
Q10: How does the structure of EDDS, compared to EDTA, contribute to its biodegradability?
A10: The presence of succinate groups in the EDDS molecule provides specific sites for enzymatic attack, facilitating its biodegradation. In contrast, EDTA lacks these susceptible sites, making it more resistant to biodegradation. []
Q11: What is the stability of EDDS under different pH conditions?
A11: The stability of EDDS can be influenced by pH. Studies have shown that the efficiency of EDDS in removing pollutants through photo-Fenton processes can vary depending on the pH, with optimal performance observed under specific pH ranges. [, , ]
Q12: Is EDDS considered a safer alternative to traditional chelating agents like EDTA in terms of environmental impact?
A12: EDDS is often considered a more environmentally friendly option due to its biodegradability. Studies have shown that it degrades faster in the environment than EDTA, reducing the risk of heavy metal mobilization and leaching. [, , , , ]
Q13: Which analytical techniques are commonly employed to quantify EDDS and heavy metal concentrations in environmental samples?
A13: Atomic Absorption Spectrometry (AAS) is widely used to determine heavy metal concentrations in various matrices, including plant tissues and soil extracts, in studies investigating EDDS-assisted phytoextraction. [, ]
Q14: What are the environmental advantages of using EDDS over traditional chelating agents like EDTA?
A14: EDDS offers several environmental advantages over EDTA, primarily due to its biodegradable nature. Studies have shown that EDDS degrades faster in the environment, minimizing the risk of persistent heavy metal complexes and potential leaching into groundwater. [, , , , ]
Q15: How does the solubility of EDDS in water compare to other chelating agents, and how does this affect its application?
A15: EDDS exhibits good solubility in water, which is a desirable property for its application in soil remediation and water treatment. This solubility facilitates the formation of complexes with heavy metal ions in solution, enhancing their removal from contaminated media. [, , ]
Q16: How does the biodegradability of EDDS compare to EDTA in different environmental conditions?
A16: EDDS generally demonstrates superior biodegradability compared to EDTA in various environmental conditions. Studies highlight that the presence of certain microorganisms and appropriate environmental factors accelerate the breakdown of EDDS, reducing its persistence in the environment. [, , , ]
Q17: Are there any other biodegradable chelating agents being explored as alternatives to EDDS for environmental remediation?
A17: Yes, researchers are investigating other biodegradable chelating agents like nitrilotriacetic acid (NTA) and citric acid (CA) as potential alternatives to EDDS. While these chelators show promise, their effectiveness can vary depending on the specific metal contaminant and environmental conditions. [, , ]
Q18: Are there any strategies to recover and recycle EDDS after its use in soil remediation or water treatment processes?
A18: Research on EDDS recycling is ongoing. Some studies explore the use of ion exchange resins to recover EDDS from treated solutions, allowing for its potential reuse and reducing the overall environmental footprint of remediation processes. [, ]
Disclaimer and Information on In-Vitro Research Products
Please be aware that all articles and product information presented on BenchChem are intended solely for informational purposes. The products available for purchase on BenchChem are specifically designed for in-vitro studies, which are conducted outside of living organisms. In-vitro studies, derived from the Latin term "in glass," involve experiments performed in controlled laboratory settings using cells or tissues. It is important to note that these products are not categorized as medicines or drugs, and they have not received approval from the FDA for the prevention, treatment, or cure of any medical condition, ailment, or disease. We must emphasize that any form of bodily introduction of these products into humans or animals is strictly prohibited by law. It is essential to adhere to these guidelines to ensure compliance with legal and ethical standards in research and experimentation.