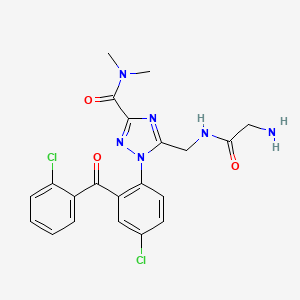
Rilmazafone
Overview
Description
Rilmazafone is a water-soluble prodrug developed in Japan, primarily used for its sedative and hypnotic effects. It is converted into active benzodiazepine metabolites within the human body, which then exert the desired pharmacological effects . Unlike traditional benzodiazepines, this compound itself does not contain a benzene ring fused with a diazepine ring, making it unique in its class .
Mechanism of Action
Target of Action
Rilmazafone is a prodrug that is metabolized in the human body into several benzodiazepine metabolites . These metabolites are the active compounds that interact with the body’s benzodiazepine receptors .
Mode of Action
Once inside the body, it is metabolized by aminopeptidase enzymes in the small intestine to form the principal active benzodiazepine rilmazolam . This metabolite then interacts with the benzodiazepine receptors in the body, leading to sedative and hypnotic effects .
Biochemical Pathways
The biochemical pathway involved in the action of this compound is the metabolism of the prodrug into its active benzodiazepine metabolites. This process involves a ring closure resulting in a triazolo benzodiazepine structure similar to alprazolam . The metabolites produced include rilmazolam, N-desmethyl rilmazolam, and di-desmethyl rilmazolam .
Pharmacokinetics
This compound is a water-soluble prodrug , which aids in its absorption in the body. After oral administration, it is metabolized in the body to form its active metabolites . The elimination half-life of this compound is approximately 10.5 hours , and it is excreted in the urine .
Result of Action
The active metabolites of this compound interact with the benzodiazepine receptors in the body, leading to sedative and hypnotic effects . This results in the reduction of anxiety, induction of sleep, and muscle relaxation .
Action Environment
The action of this compound can be influenced by various environmental factors. For instance, the presence of other substances in the body can affect the metabolism and action of this compound. In certain cases, the intake of this compound was found to be the cause of death, with additional toxicological findings including medications such as haloperidol, alimemazine, fluoxetine, olanzapine, and acetaminophen . Therefore, the environment in which this compound is used, including the presence of other substances, can significantly influence its action, efficacy, and stability.
Biochemical Analysis
Biochemical Properties
Rilmazafone metabolizes into active compounds by a ring closure, resulting in a triazolo benzodiazepine structure similar to alprazolam . This process involves the interaction with various enzymes and proteins, including aminopeptidase enzymes in the small intestine .
Cellular Effects
This compound itself has no effects on benzodiazepine receptors nor does it produce any psychoactive effects prior to metabolism . Once metabolized, the active benzodiazepine metabolites can influence various cellular processes, including cell signaling pathways and gene expression .
Molecular Mechanism
This compound is metabolized by aminopeptidase enzymes in the small intestine to form the principal active benzodiazepine rilmazolam . This process involves binding interactions with these enzymes, leading to changes in gene expression and enzyme activation or inhibition .
Temporal Effects in Laboratory Settings
The effects of this compound and its metabolites have been studied over time in laboratory settings . These studies have provided information on the product’s stability, degradation, and long-term effects on cellular function .
Metabolic Pathways
This compound is involved in metabolic pathways that result in the formation of several active compounds . This process involves a ring closure resulting in a triazolo benzodiazepine structure similar to alprazolam .
Subcellular Localization
Given that it is metabolized by enzymes in the small intestine, it is likely that it is localized to this region prior to metabolism .
Preparation Methods
The synthesis of rilmazafone involves several steps, starting with the preparation of the core triazole structure. The synthetic route typically includes the following steps:
Formation of the triazole ring: This involves the reaction of appropriate precursors under controlled conditions to form the triazole ring.
Introduction of the benzophenone moiety: This step involves the attachment of the benzophenone group to the triazole ring.
Final modifications:
Industrial production methods for this compound are not extensively documented, but they likely follow similar synthetic routes with optimizations for large-scale production.
Chemical Reactions Analysis
Rilmazafone undergoes several chemical reactions, primarily during its metabolism in the human body:
Ring closure: The hydrolyzed product undergoes a ring closure reaction to form the active benzodiazepine structure.
Oxidation and reduction: These reactions may occur during the metabolic process, leading to the formation of various metabolites.
Common reagents and conditions used in these reactions include aminopeptidase enzymes and physiological conditions within the human body. The major products formed from these reactions are active benzodiazepine metabolites such as rilmazolam .
Scientific Research Applications
Rilmazafone has several scientific research applications, including:
Pharmacological studies: It is used to study the pharmacokinetics and pharmacodynamics of benzodiazepine prodrugs.
Toxicological research: This compound is studied for its potential toxic effects and its involvement in fatal intoxications.
Clinical research: It is used in clinical studies to evaluate its efficacy and safety as a sedative and hypnotic agent.
Forensic science: This compound and its metabolites are analyzed in forensic toxicology to identify cases of drug abuse and intoxication.
Comparison with Similar Compounds
Rilmazafone is unique compared to other benzodiazepines due to its prodrug nature and lack of a benzene ring fused with a diazepine ring. Similar compounds include:
Rilmazolam: The active metabolite of this compound, which contains the benzodiazepine ring structure.
Alprazolam: A traditional benzodiazepine with a similar triazolo benzodiazepine structure.
Pagoclone: Another compound that undergoes similar metabolic transformations.
This compound’s uniqueness lies in its prodrug nature, which allows it to be converted into active metabolites within the body, providing a controlled release of the active benzodiazepine .
Properties
IUPAC Name |
5-[[(2-aminoacetyl)amino]methyl]-1-[4-chloro-2-(2-chlorobenzoyl)phenyl]-N,N-dimethyl-1,2,4-triazole-3-carboxamide | |
---|---|---|
Source | PubChem | |
URL | https://pubchem.ncbi.nlm.nih.gov | |
Description | Data deposited in or computed by PubChem | |
InChI |
InChI=1S/C21H20Cl2N6O3/c1-28(2)21(32)20-26-17(11-25-18(30)10-24)29(27-20)16-8-7-12(22)9-14(16)19(31)13-5-3-4-6-15(13)23/h3-9H,10-11,24H2,1-2H3,(H,25,30) | |
Source | PubChem | |
URL | https://pubchem.ncbi.nlm.nih.gov | |
Description | Data deposited in or computed by PubChem | |
InChI Key |
KYHFRCPLIGODFH-UHFFFAOYSA-N | |
Source | PubChem | |
URL | https://pubchem.ncbi.nlm.nih.gov | |
Description | Data deposited in or computed by PubChem | |
Canonical SMILES |
CN(C)C(=O)C1=NN(C(=N1)CNC(=O)CN)C2=C(C=C(C=C2)Cl)C(=O)C3=CC=CC=C3Cl | |
Source | PubChem | |
URL | https://pubchem.ncbi.nlm.nih.gov | |
Description | Data deposited in or computed by PubChem | |
Molecular Formula |
C21H20Cl2N6O3 | |
Source | PubChem | |
URL | https://pubchem.ncbi.nlm.nih.gov | |
Description | Data deposited in or computed by PubChem | |
Related CAS |
85815-37-8 (hydrochloride) | |
Record name | Rilmazafone [INN] | |
Source | ChemIDplus | |
URL | https://pubchem.ncbi.nlm.nih.gov/substance/?source=chemidplus&sourceid=0099593256 | |
Description | ChemIDplus is a free, web search system that provides access to the structure and nomenclature authority files used for the identification of chemical substances cited in National Library of Medicine (NLM) databases, including the TOXNET system. | |
DSSTOX Substance ID |
DTXSID10244150 | |
Record name | Rilmazafone | |
Source | EPA DSSTox | |
URL | https://comptox.epa.gov/dashboard/DTXSID10244150 | |
Description | DSSTox provides a high quality public chemistry resource for supporting improved predictive toxicology. | |
Molecular Weight |
475.3 g/mol | |
Source | PubChem | |
URL | https://pubchem.ncbi.nlm.nih.gov | |
Description | Data deposited in or computed by PubChem | |
CAS No. |
99593-25-6 | |
Record name | 5-[[(2-Aminoacetyl)amino]methyl]-1-[4-chloro-2-(2-chlorobenzoyl)phenyl]-N,N-dimethyl-1H-1,2,4-triazole-3-carboxamide | |
Source | CAS Common Chemistry | |
URL | https://commonchemistry.cas.org/detail?cas_rn=99593-25-6 | |
Description | CAS Common Chemistry is an open community resource for accessing chemical information. Nearly 500,000 chemical substances from CAS REGISTRY cover areas of community interest, including common and frequently regulated chemicals, and those relevant to high school and undergraduate chemistry classes. This chemical information, curated by our expert scientists, is provided in alignment with our mission as a division of the American Chemical Society. | |
Explanation | The data from CAS Common Chemistry is provided under a CC-BY-NC 4.0 license, unless otherwise stated. | |
Record name | Rilmazafone [INN] | |
Source | ChemIDplus | |
URL | https://pubchem.ncbi.nlm.nih.gov/substance/?source=chemidplus&sourceid=0099593256 | |
Description | ChemIDplus is a free, web search system that provides access to the structure and nomenclature authority files used for the identification of chemical substances cited in National Library of Medicine (NLM) databases, including the TOXNET system. | |
Record name | Rilmazafone | |
Source | EPA DSSTox | |
URL | https://comptox.epa.gov/dashboard/DTXSID10244150 | |
Description | DSSTox provides a high quality public chemistry resource for supporting improved predictive toxicology. | |
Record name | RILMAZAFONE | |
Source | FDA Global Substance Registration System (GSRS) | |
URL | https://gsrs.ncats.nih.gov/ginas/app/beta/substances/CU3H37T766 | |
Description | The FDA Global Substance Registration System (GSRS) enables the efficient and accurate exchange of information on what substances are in regulated products. Instead of relying on names, which vary across regulatory domains, countries, and regions, the GSRS knowledge base makes it possible for substances to be defined by standardized, scientific descriptions. | |
Explanation | Unless otherwise noted, the contents of the FDA website (www.fda.gov), both text and graphics, are not copyrighted. They are in the public domain and may be republished, reprinted and otherwise used freely by anyone without the need to obtain permission from FDA. Credit to the U.S. Food and Drug Administration as the source is appreciated but not required. | |
Retrosynthesis Analysis
AI-Powered Synthesis Planning: Our tool employs the Template_relevance Pistachio, Template_relevance Bkms_metabolic, Template_relevance Pistachio_ringbreaker, Template_relevance Reaxys, Template_relevance Reaxys_biocatalysis model, leveraging a vast database of chemical reactions to predict feasible synthetic routes.
One-Step Synthesis Focus: Specifically designed for one-step synthesis, it provides concise and direct routes for your target compounds, streamlining the synthesis process.
Accurate Predictions: Utilizing the extensive PISTACHIO, BKMS_METABOLIC, PISTACHIO_RINGBREAKER, REAXYS, REAXYS_BIOCATALYSIS database, our tool offers high-accuracy predictions, reflecting the latest in chemical research and data.
Strategy Settings
Precursor scoring | Relevance Heuristic |
---|---|
Min. plausibility | 0.01 |
Model | Template_relevance |
Template Set | Pistachio/Bkms_metabolic/Pistachio_ringbreaker/Reaxys/Reaxys_biocatalysis |
Top-N result to add to graph | 6 |
Feasible Synthetic Routes
Disclaimer and Information on In-Vitro Research Products
Please be aware that all articles and product information presented on BenchChem are intended solely for informational purposes. The products available for purchase on BenchChem are specifically designed for in-vitro studies, which are conducted outside of living organisms. In-vitro studies, derived from the Latin term "in glass," involve experiments performed in controlled laboratory settings using cells or tissues. It is important to note that these products are not categorized as medicines or drugs, and they have not received approval from the FDA for the prevention, treatment, or cure of any medical condition, ailment, or disease. We must emphasize that any form of bodily introduction of these products into humans or animals is strictly prohibited by law. It is essential to adhere to these guidelines to ensure compliance with legal and ethical standards in research and experimentation.