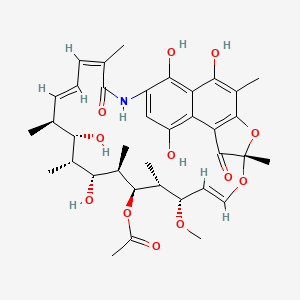
Rifamycin
Overview
Description
Rifamycin is a group of antibiotics that are synthesized either naturally by the bacterium Amycolatopsis rifamycinica or artificially. They belong to the larger family of ansamycins and are particularly effective against mycobacteria. This compound is used to treat diseases such as tuberculosis, leprosy, and mycobacterium avium complex infections . The compound was first isolated in 1957 from a fermentation culture of Streptomyces mediterranei .
Mechanism of Action
Target of Action
Rifamycin primarily targets the DNA-dependent RNA polymerase of prokaryotes . This enzyme plays a crucial role in the transcription process, where it synthesizes RNA from a DNA template .
Mode of Action
This compound interacts with its target by strongly binding to the DNA-dependent RNA polymerase . This binding occurs deep within the DNA/RNA channel of the polymerase subunit, effectively blocking the elongation of RNA . This inhibition of RNA synthesis is thought to be related to the initiation phase of the process .
Biochemical Pathways
This compound’s action affects the RNA synthesis pathway in bacteria . By inhibiting RNA polymerase, it disrupts the transcription process, preventing the production of essential proteins and thereby inhibiting bacterial growth . The exact downstream effects of this disruption depend on the specific bacterial species and the proteins that are affected.
Pharmacokinetics
This compound exhibits considerable inter- and intra-individual variability in its pharmacokinetics . It is known to induce its own clearance through the liver (autoinduction) via its effects on metabolizing enzymes, leading to a decrease in this compound exposure during the first 6–14 days of oral administration . This effect can lead to reduced bioavailability and enhanced clearance of some coadministered medications . Rifampicin, a derivative of this compound, is about 80% protein-bound to albumin and is distributed intra- and extracellularly due to its polar nature .
Result of Action
The primary result of this compound’s action is the suppression of bacterial growth . By inhibiting the production of RNA, this compound prevents bacteria from synthesizing the proteins they need to grow and reproduce . This leads to the death of the bacteria, making this compound an effective antibacterial agent .
Action Environment
The action of this compound can be influenced by various environmental factors. For instance, the presence of food can reduce the absorption of this compound, leading to lower drug concentrations in the body . Additionally, certain conditions such as malnutrition, HIV infection, diabetes mellitus, and hepatic cirrhosis can alter this compound exposure and/or efficacy . Furthermore, the development of bacterial resistance to this compound can also influence its action and efficacy .
Biochemical Analysis
Biochemical Properties
Rifamycin plays a crucial role in biochemical reactions by inhibiting bacterial DNA-dependent RNA polymerase. This inhibition prevents the transcription of bacterial DNA into RNA, effectively halting protein synthesis and leading to bacterial cell death . This compound interacts with several biomolecules, including enzymes and proteins. It binds specifically to the beta subunit of RNA polymerase, forming a stable complex that obstructs the elongation of the RNA chain . This interaction is highly selective, ensuring that this compound targets bacterial cells without affecting human cells.
Cellular Effects
This compound exerts significant effects on various types of cells and cellular processes. In bacterial cells, it rapidly kills fast-dividing strains as well as “persister” cells, which remain dormant and evade antibiotic activity . This dual action makes this compound particularly effective against chronic infections. Additionally, this compound influences cell signaling pathways and gene expression by inhibiting RNA synthesis, leading to a cascade of effects that disrupt cellular metabolism and function .
Molecular Mechanism
At the molecular level, this compound’s mechanism of action involves binding to the DNA-dependent RNA polymerase of prokaryotes. This binding inhibits the enzyme’s activity, preventing the transcription of DNA into RNA . The inhibition is achieved through the formation of a stable complex between this compound and the beta subunit of RNA polymerase, which blocks the elongation of the RNA chain . This selective inhibition ensures that this compound effectively targets bacterial cells while minimizing effects on human cells.
Temporal Effects in Laboratory Settings
In laboratory settings, the effects of this compound have been observed to change over time. This compound is known for its stability and sustained activity, making it effective in long-term treatments . Resistance can develop over time due to mutations in the RNA polymerase gene, which reduce the binding affinity of this compound . Studies have shown that this compound maintains its antibacterial activity for extended periods, but its effectiveness can diminish with prolonged use due to the emergence of resistant bacterial strains .
Dosage Effects in Animal Models
The effects of this compound vary with different dosages in animal models. At therapeutic doses, this compound effectively eliminates bacterial infections without causing significant adverse effects . At high doses, this compound can cause toxic effects, including hepatotoxicity and gastrointestinal disturbances . Studies in animal models have shown that the therapeutic window for this compound is relatively wide, allowing for effective treatment with minimal risk of toxicity .
Metabolic Pathways
This compound is metabolized primarily in the liver, where it undergoes biotransformation to several active metabolites . These metabolites are excreted mainly in bile and, to a lesser extent, in urine . This compound induces the cytochrome P450 enzyme system, particularly CYP3A4, which can lead to interactions with other drugs metabolized by this pathway . This induction can affect the metabolic flux and levels of various metabolites, influencing the overall pharmacokinetics of this compound .
Transport and Distribution
This compound is well-absorbed orally and widely distributed in body tissues and fluids, including cerebrospinal fluid . It is concentrated in polymorphonuclear granulocytes and macrophages, facilitating the clearance of bacteria from abscesses . This compound is transported within cells by binding to plasma proteins, which aids in its distribution to various tissues . Its high lipid solubility allows it to penetrate cell membranes and reach intracellular targets effectively .
Subcellular Localization
This compound’s subcellular localization is primarily within the cytoplasm, where it exerts its antibacterial effects by targeting RNA polymerase . It does not require specific targeting signals or post-translational modifications to reach its site of action. Instead, its high affinity for bacterial RNA polymerase ensures that it localizes to the appropriate subcellular compartment to inhibit RNA synthesis .
Preparation Methods
Synthetic Routes and Reaction Conditions: Rifamycin can be synthesized through various methods. One common method involves the reaction of this compound S with tert-butylamine in a microreactor, resulting in rifampicin with a 67% overall yield . Another method involves the preparation of this compound sodium injection, which includes steps such as dissolving the raw material in water for injection, adjusting the pH, and filtering the solution .
Industrial Production Methods: Industrial production of this compound often involves fermentation processes using Amycolatopsis rifamycinica. The fermentation culture is carefully monitored and controlled to optimize the yield of this compound. Continuous flow synthesis methods are also being explored to reduce costs and improve efficiency .
Chemical Reactions Analysis
Types of Reactions: Rifamycin undergoes various chemical reactions, including oxidation, reduction, and substitution. For example, the conversion of this compound SV to rifampicin involves the reaction with 1-amino-4-methyl piperazine .
Common Reagents and Conditions: Common reagents used in the synthesis of this compound derivatives include tert-butylamine, 1-amino-4-methyl piperazine, and sodium metabisulfite. Reaction conditions often involve controlled pH, temperature, and the use of microreactors for continuous flow synthesis .
Major Products: Major products formed from these reactions include rifampicin, rifabutin, rifapentine, and rifaximin. These derivatives have slight differences in their physicochemical properties but share the same core structure .
Scientific Research Applications
Rifamycin has a wide range of scientific research applications:
Chemistry: Used as a model compound for studying antibiotic synthesis and mechanisms.
Biology: Studied for its effects on bacterial RNA polymerase and its role in inhibiting bacterial growth.
Medicine: Widely used in the treatment of tuberculosis, leprosy, and other bacterial infections.
Industry: Employed in the production of various this compound derivatives for pharmaceutical use.
Comparison with Similar Compounds
- Rifampicin
- Rifabutin
- Rifapentine
- Rifaximin
Comparison: Rifamycin and its derivatives share a common mechanism of action but differ in their pharmacokinetic properties and clinical uses. For example, rifampicin is widely used for tuberculosis treatment, while rifaximin is used for treating traveler’s diarrhea . Rifabutin and rifapentine have different absorption rates and are used for specific bacterial infections . The unique structure of this compound allows for modifications that enhance its efficacy and reduce resistance .
This compound stands out due to its broad-spectrum activity and its ability to inhibit bacterial RNA polymerase without cross-resistance with other antibiotics .
Properties
Key on ui mechanism of action |
Rifamycins, as well as all the other members of this group, present an antibacterial mechanism of action related to the inhibition of RNA synthesis. This mechanism of action is done by the strong binding to the DNA-dependent RNA polymerase of prokaryotes. The inhibition of the RNA synthesis is thought to be related with the initiation phase of the process and to involve stacking interactions between the naphthalene ring and the aromatic moiety in the polymerase. As well, it has been suggested that the presence of zinc atoms in the polymerase allows for the binding of phenolic -OH groups of the naphthalene ring. In eukaryotic cells, the binding is significantly reduced making them at least 100 to 10,000 times less sensitive to the action of rifamycins. The members of the rifamycin family present the same mechanism of action and the structural modifications are usually related to pharmacokinetic properties as well as to the interaction with eukaryotic cells. |
---|---|
CAS No. |
6998-60-3 |
Molecular Formula |
C37H47NO12 |
Molecular Weight |
697.8 g/mol |
IUPAC Name |
[(7S,11S,12R,13S,14R,15R,16R,17S,18S,19Z,21Z)-2,15,17,27,29-pentahydroxy-11-methoxy-3,7,12,14,16,18,22-heptamethyl-6,23-dioxo-8,30-dioxa-24-azatetracyclo[23.3.1.14,7.05,28]triaconta-1(29),2,4,9,19,21,25,27-octaen-13-yl] acetate |
InChI |
InChI=1S/C37H47NO12/c1-16-11-10-12-17(2)36(46)38-23-15-24(40)26-27(32(23)44)31(43)21(6)34-28(26)35(45)37(8,50-34)48-14-13-25(47-9)18(3)33(49-22(7)39)20(5)30(42)19(4)29(16)41/h10-16,18-20,25,29-30,33,40-44H,1-9H3,(H,38,46)/b11-10-,14-13?,17-12-/t16-,18+,19+,20+,25-,29-,30+,33+,37-/m0/s1 |
InChI Key |
HJYYPODYNSCCOU-PTWHBISLSA-N |
SMILES |
CC1C=CC=C(C(=O)NC2=CC(=C3C(=C2O)C(=C(C4=C3C(=O)C(O4)(OC=CC(C(C(C(C(C(C1O)C)O)C)OC(=O)C)C)OC)C)C)O)O)C |
Isomeric SMILES |
C[C@H]1/C=C\C=C(/C(=O)NC2=CC(=C3C(=C2O)C(=C(C4=C3C(=O)[C@](O4)(OC=C[C@@H]([C@H]([C@H]([C@@H]([C@@H]([C@@H]([C@H]1O)C)O)C)OC(=O)C)C)OC)C)C)O)O)\C |
Canonical SMILES |
CC1C=CC=C(C(=O)NC2=CC(=C3C(=C2O)C(=C(C4=C3C(=O)C(O4)(OC=CC(C(C(C(C(C(C1O)C)O)C)OC(=O)C)C)OC)C)C)O)O)C |
Appearance |
Solid powder |
boiling_point |
972.8 ºC at 760 mm Hg |
melting_point |
171 ºC 300.0 °C |
Key on ui other cas no. |
6998-60-3 |
Purity |
>98% (or refer to the Certificate of Analysis) |
Related CAS |
14897-39-3 (unspecified hydrochloride salt) 15105-92-7 (mono-hydrochloride salt) 6998-60-3 (Parent) |
shelf_life |
>3 years if stored properly |
solubility |
Insoluble |
storage |
Dry, dark and at 0 - 4 C for short term (days to weeks) or -20 C for long term (months to years). |
Synonyms |
5,6,9,17,19,21-hexahydroxy-23-methoxy-2,4,12,16,18,20,22-heptamethyl-2,7-(epoxypentadeca(1,11,13)trienimino)naphtho(2,1-b)furan-1,11(2-H)-dione, 21-acetate Aemcolo Otafa R-75-1 Rifacin Rifamicina Colirio rifamycin SV rifamycin SV, monosodium salt rifamycin SV, sodium salt Rifamycine Chibret Rifocina Rifocyna |
Origin of Product |
United States |
Retrosynthesis Analysis
AI-Powered Synthesis Planning: Our tool employs the Template_relevance Pistachio, Template_relevance Bkms_metabolic, Template_relevance Pistachio_ringbreaker, Template_relevance Reaxys, Template_relevance Reaxys_biocatalysis model, leveraging a vast database of chemical reactions to predict feasible synthetic routes.
One-Step Synthesis Focus: Specifically designed for one-step synthesis, it provides concise and direct routes for your target compounds, streamlining the synthesis process.
Accurate Predictions: Utilizing the extensive PISTACHIO, BKMS_METABOLIC, PISTACHIO_RINGBREAKER, REAXYS, REAXYS_BIOCATALYSIS database, our tool offers high-accuracy predictions, reflecting the latest in chemical research and data.
Strategy Settings
Precursor scoring | Relevance Heuristic |
---|---|
Min. plausibility | 0.01 |
Model | Template_relevance |
Template Set | Pistachio/Bkms_metabolic/Pistachio_ringbreaker/Reaxys/Reaxys_biocatalysis |
Top-N result to add to graph | 6 |
Feasible Synthetic Routes
Q1: How do rifamycins exert their antibacterial activity?
A1: Rifamycins specifically bind to the β-subunit of bacterial DNA-dependent RNA polymerase (RNAP) [, , , ]. This binding inhibits the initiation of RNA synthesis, effectively halting bacterial growth and leading to cell death [, , ].
Q2: Can rifamycins inhibit RNAP in organisms other than bacteria?
A2: While highly effective against bacterial RNAP, rifamycins demonstrate limited activity against mammalian RNAP, contributing to their selective toxicity []. There are reports of rifamycins inhibiting the reverse transcriptase (RT) of some RNA viruses [].
Q3: What are the essential structural features of rifamycins responsible for their activity?
A3: Rifamycins require a naphthalene ring with oxygen atoms at specific positions, two unsubstituted hydroxyl groups on the ansa chain, and a precise spatial arrangement of oxygen atoms for optimal activity []. The ansa bridge links non-adjacent positions of the aromatic nucleus [].
Q4: Which structural modifications of rifamycins have been explored to enhance their activity?
A4: Most modifications focus on the C-3 or C-4 positions of the naphthoquinone/naphthohydroquinone chromophore, leading to derivatives like rifampicin []. One example is the addition of a benzoxazine ring, yielding benzoxazinorifamycins like rifalazil, which exhibit improved activity against rifamycin-resistant strains [, , ].
Q5: How do bacteria develop resistance to rifamycins?
A5: The most common mechanism involves mutations in the this compound resistance-determining region (RRDR) within the rpoB gene encoding the β-subunit of RNAP [, ]. These mutations alter the drug's binding site, reducing its efficacy [, ].
Q6: Are there enzymes that contribute to this compound resistance?
A6: Yes, some bacteria possess enzymes that inactivate rifamycins. One example is the ADP-ribosyltransferase Arr, found in Mycobacterium abscessus, which adds ADP-ribose to rifampicin, rendering it ineffective [, ]. Another mechanism involves enzymatic degradation of rifamycins by Rox enzymes, a type of flavin monooxygenase [].
Q7: Is there cross-resistance between rifamycins and other antibiotic classes?
A8: Fidaxomicin, another RNAP inhibitor, demonstrates a lower propensity for the development of resistance to rifamycins and exhibits no cross-resistance with rifamycins, highlighting differences in their mechanisms of action [].
Q8: How is the this compound scaffold biosynthesized?
A9: The core structure is assembled by a type I modular polyketide synthase (PKS) using 3-amino-5-hydroxybenzoic acid (AHBA), acetate, and propionate as building blocks []. The polyketide framework of this compound B is assembled from 3-amino-5-hydroxybenzoic acid (AHBA), two molecules of acetate, and eight molecules of propionate [].
Q9: What are some of the key intermediates in the this compound biosynthetic pathway?
A10: Proansamycin X is a proposed early macrocyclic intermediate []. This compound W is a predominant intermediate that undergoes significant structural rearrangements to form this compound B [, ]. Studies on mutants with inactivated rifF gene provide insights into the timing of naphthoquinone ring formation and other biosynthetic steps [].
Q10: What is the role of this compound oxidase in this compound production?
A11: this compound oxidase catalyzes the biotransformation of the less active this compound B to the more potent this compound S, a key intermediate for synthesizing various semi-synthetic rifamycins [, ].
Q11: How can the production of this compound B be improved?
A11: Several approaches have been explored, including:
- Strain Optimization: Selecting for high-yielding strains based on colony morphology and implementing genetic modifications, such as gene amplification [, ].
- Media Optimization: Replacing ammonium sulfate with potassium nitrate or ammonium nitrate as the nitrogen source can significantly enhance production [, ].
- Feeding Strategies: Implementing a fed-batch fermentation process with optimized feeding of glucose, yeast extract, and nitrogen sources can further improve this compound B yields [].
Q12: What are the clinical applications of rifamycins?
A12: Rifamycins are crucial for treating infections caused by:
- Mycobacterium tuberculosis (tuberculosis) [, , , , ]
- Mycobacterium leprae (leprosy) []
- AIDS-related mycobacterial infections [, , ]
- Staphylococcus aureus infections [, , ]
- Streptococcus pyogenes infections []
Q13: How do rifamycins behave within the body?
A14: The pharmacokinetic properties of rifamycins are complex, leading to significant inter-individual variability in drug exposure []. Factors like body weight, disease state, food intake, and genetic polymorphisms in drug transporter proteins can influence their absorption, distribution, metabolism, and excretion [].
Q14: What is the current research focus on improving this compound therapy?
A14: Current research focuses on:
- Optimizing Dosing: Exploring higher doses to shorten TB treatment duration and investigating the relationship between this compound dose and toxicity [].
- Developing New Analogs: Creating derivatives with enhanced activity against resistant strains, improved pharmacokinetic properties, and reduced toxicity [, , , ].
- Exploring Drug Synergies: Investigating this compound combinations with other antibiotics or host-directed therapies to enhance efficacy and combat resistance [].
Disclaimer and Information on In-Vitro Research Products
Please be aware that all articles and product information presented on BenchChem are intended solely for informational purposes. The products available for purchase on BenchChem are specifically designed for in-vitro studies, which are conducted outside of living organisms. In-vitro studies, derived from the Latin term "in glass," involve experiments performed in controlled laboratory settings using cells or tissues. It is important to note that these products are not categorized as medicines or drugs, and they have not received approval from the FDA for the prevention, treatment, or cure of any medical condition, ailment, or disease. We must emphasize that any form of bodily introduction of these products into humans or animals is strictly prohibited by law. It is essential to adhere to these guidelines to ensure compliance with legal and ethical standards in research and experimentation.