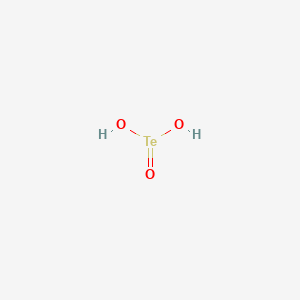
Tellurous acid
Overview
Description
Tellurous acid is an inorganic compound with the chemical formula H₂TeO₃. It is the oxoacid of tellurium (IV) and is known for its colorless crystalline appearance. This compound is relatively less characterized compared to other oxoacids of tellurium. This compound is formed by the hydrolysis of tellurium dioxide with water .
Preparation Methods
Synthetic Routes and Reaction Conditions: Tellurous acid can be synthesized in a laboratory setting by the controlled reaction of tellurium with potent oxidizing agents. The most common method involves the oxidation of tellurium with nitric acid, which leads to the formation of this compound. This process must be conducted with great care, as the reaction is highly exothermic and can easily lead to the formation of other tellurium oxides if not properly controlled .
Industrial Production Methods: it can be prepared by treating tellurium dioxide with water under controlled conditions .
Chemical Reactions Analysis
Types of Reactions: Tellurous acid undergoes various chemical reactions, including oxidation, reduction, and substitution processes.
Common Reagents and Conditions:
Oxidation: this compound can be oxidized by hydrogen peroxide to form tellurium dioxide (TeO₂) and water.
Reduction: It can be reduced to elemental tellurium using reducing agents such as sulfur dioxide.
Substitution: this compound can react with bases to form tellurite salts.
Major Products Formed:
Oxidation: Tellurium dioxide (TeO₂)
Reduction: Elemental tellurium (Te)
Substitution: Tellurite salts (e.g., potassium tellurite, K₂TeO₃)
Scientific Research Applications
Tellurous acid has various applications in scientific research, particularly in the field of chemistry. It serves as a key starting material in the synthesis of other tellurium compounds. Additionally, it has been utilized in various types of chemical reactions as an oxidizing agent . In industry, this compound is used in the production of tellurium salts and as a reagent in chemical synthesis .
Mechanism of Action
The mechanism of action of tellurous acid involves its ability to undergo oxidation and reduction reactions. In biological systems, tellurium compounds can interact with thiol groups in proteins, leading to the disruption of cellular functions. This interaction is believed to be the basis for its antibacterial and anticancer properties . The exact molecular targets and pathways involved in these effects are still under investigation.
Comparison with Similar Compounds
Selenous acid (H₂SeO₃): Similar to tellurous acid, selenous acid is an oxoacid of selenium (IV) and exhibits similar chemical properties.
Sulfurous acid (H₂SO₃): This is the oxoacid of sulfur (IV) and is more stable compared to this compound.
Uniqueness: this compound is unique due to its relatively less stable nature and its specific applications in the synthesis of tellurium compounds. Unlike sulfurous acid, which is widely used in industry, this compound has more specialized applications .
Properties
IUPAC Name |
tellurous acid | |
---|---|---|
Source | PubChem | |
URL | https://pubchem.ncbi.nlm.nih.gov | |
Description | Data deposited in or computed by PubChem | |
InChI |
InChI=1S/H2O3Te/c1-4(2)3/h(H2,1,2,3) | |
Source | PubChem | |
URL | https://pubchem.ncbi.nlm.nih.gov | |
Description | Data deposited in or computed by PubChem | |
InChI Key |
SITVSCPRJNYAGV-UHFFFAOYSA-N | |
Source | PubChem | |
URL | https://pubchem.ncbi.nlm.nih.gov | |
Description | Data deposited in or computed by PubChem | |
Canonical SMILES |
O[Te](=O)O | |
Source | PubChem | |
URL | https://pubchem.ncbi.nlm.nih.gov | |
Description | Data deposited in or computed by PubChem | |
Molecular Formula |
H2TeO3, H2O3Te | |
Record name | tellurous acid | |
Source | Wikipedia | |
URL | https://en.wikipedia.org/wiki/Tellurous_acid | |
Description | Chemical information link to Wikipedia. | |
Source | PubChem | |
URL | https://pubchem.ncbi.nlm.nih.gov | |
Description | Data deposited in or computed by PubChem | |
Related CAS |
15852-22-9 (TeO3(-2)), 22451-06-5 (di-hydrochloride salt pentahydrate) | |
Record name | Tellurous acid | |
Source | ChemIDplus | |
URL | https://pubchem.ncbi.nlm.nih.gov/substance/?source=chemidplus&sourceid=0010049237 | |
Description | ChemIDplus is a free, web search system that provides access to the structure and nomenclature authority files used for the identification of chemical substances cited in National Library of Medicine (NLM) databases, including the TOXNET system. | |
DSSTOX Substance ID |
DTXSID90143339 | |
Record name | Tellurous acid | |
Source | EPA DSSTox | |
URL | https://comptox.epa.gov/dashboard/DTXSID90143339 | |
Description | DSSTox provides a high quality public chemistry resource for supporting improved predictive toxicology. | |
Molecular Weight |
177.6 g/mol | |
Source | PubChem | |
URL | https://pubchem.ncbi.nlm.nih.gov | |
Description | Data deposited in or computed by PubChem | |
CAS No. |
10049-23-7 | |
Record name | Telluric acid (H2TeO3) | |
Source | CAS Common Chemistry | |
URL | https://commonchemistry.cas.org/detail?cas_rn=10049-23-7 | |
Description | CAS Common Chemistry is an open community resource for accessing chemical information. Nearly 500,000 chemical substances from CAS REGISTRY cover areas of community interest, including common and frequently regulated chemicals, and those relevant to high school and undergraduate chemistry classes. This chemical information, curated by our expert scientists, is provided in alignment with our mission as a division of the American Chemical Society. | |
Explanation | The data from CAS Common Chemistry is provided under a CC-BY-NC 4.0 license, unless otherwise stated. | |
Record name | Tellurous acid | |
Source | ChemIDplus | |
URL | https://pubchem.ncbi.nlm.nih.gov/substance/?source=chemidplus&sourceid=0010049237 | |
Description | ChemIDplus is a free, web search system that provides access to the structure and nomenclature authority files used for the identification of chemical substances cited in National Library of Medicine (NLM) databases, including the TOXNET system. | |
Record name | Tellurous acid | |
Source | EPA DSSTox | |
URL | https://comptox.epa.gov/dashboard/DTXSID90143339 | |
Description | DSSTox provides a high quality public chemistry resource for supporting improved predictive toxicology. | |
Record name | Dihydrogen trioxotellurate | |
Source | European Chemicals Agency (ECHA) | |
URL | https://echa.europa.eu/substance-information/-/substanceinfo/100.030.145 | |
Description | The European Chemicals Agency (ECHA) is an agency of the European Union which is the driving force among regulatory authorities in implementing the EU's groundbreaking chemicals legislation for the benefit of human health and the environment as well as for innovation and competitiveness. | |
Explanation | Use of the information, documents and data from the ECHA website is subject to the terms and conditions of this Legal Notice, and subject to other binding limitations provided for under applicable law, the information, documents and data made available on the ECHA website may be reproduced, distributed and/or used, totally or in part, for non-commercial purposes provided that ECHA is acknowledged as the source: "Source: European Chemicals Agency, http://echa.europa.eu/". Such acknowledgement must be included in each copy of the material. ECHA permits and encourages organisations and individuals to create links to the ECHA website under the following cumulative conditions: Links can only be made to webpages that provide a link to the Legal Notice page. | |
Record name | TELLUROUS ACID | |
Source | FDA Global Substance Registration System (GSRS) | |
URL | https://gsrs.ncats.nih.gov/ginas/app/beta/substances/IVA6SGP6QM | |
Description | The FDA Global Substance Registration System (GSRS) enables the efficient and accurate exchange of information on what substances are in regulated products. Instead of relying on names, which vary across regulatory domains, countries, and regions, the GSRS knowledge base makes it possible for substances to be defined by standardized, scientific descriptions. | |
Explanation | Unless otherwise noted, the contents of the FDA website (www.fda.gov), both text and graphics, are not copyrighted. They are in the public domain and may be republished, reprinted and otherwise used freely by anyone without the need to obtain permission from FDA. Credit to the U.S. Food and Drug Administration as the source is appreciated but not required. | |
Disclaimer and Information on In-Vitro Research Products
Please be aware that all articles and product information presented on BenchChem are intended solely for informational purposes. The products available for purchase on BenchChem are specifically designed for in-vitro studies, which are conducted outside of living organisms. In-vitro studies, derived from the Latin term "in glass," involve experiments performed in controlled laboratory settings using cells or tissues. It is important to note that these products are not categorized as medicines or drugs, and they have not received approval from the FDA for the prevention, treatment, or cure of any medical condition, ailment, or disease. We must emphasize that any form of bodily introduction of these products into humans or animals is strictly prohibited by law. It is essential to adhere to these guidelines to ensure compliance with legal and ethical standards in research and experimentation.