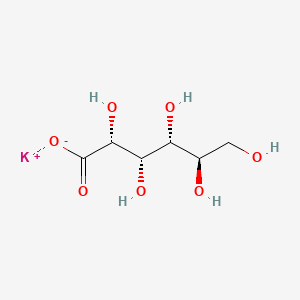
Potassium gluconate
Overview
Description
Potassium gluconate is the potassium salt of gluconic acid. It is commonly used as a dietary supplement to prevent or treat low potassium levels in the blood. Potassium is an essential mineral that plays a crucial role in maintaining the normal function of cells, nerves, muscles, and the heart. This compound is often preferred over other potassium salts due to its palatability and non-acidifying nature .
Mechanism of Action
Target of Action
Potassium gluconate is a salt of the potassium cation . Its primary target is the intracellular fluid, where it plays a key role in maintaining cell function . Potassium is an essential nutrient and the most abundant cation in the intracellular fluid .
Mode of Action
This compound interacts with its targets by entering cells via active transport from extracellular fluid . It is essential for the conduction of nerve impulses in the heart, brain, and skeletal muscle; contraction of cardiac, skeletal, and smooth muscles; maintenance of normal renal function, acid-base balance, carbohydrate metabolism, and gastric secretion .
Pharmacokinetics
This compound is well absorbed from the upper gastrointestinal tract . After absorption, it enters cells via active transport from extracellular fluid . It is primarily excreted through urine, with small amounts also excreted through the skin and feces . Most intestinal potassium is reabsorbed .
Result of Action
The action of this compound results in the maintenance of normal cell function, especially in excitable cells such as skeletal muscles, the heart, and nerves . It helps prevent hypokalemia (low potassium levels) in patients who would be at particular risk if hypokalemia were to develop . In addition to serving as a preventative supplement, this compound also serves as a treatment for decreased potassium levels .
Action Environment
The action, efficacy, and stability of this compound can be influenced by various environmental factors. For instance, the rate of potassium intake can affect the efficacy of this compound. If the rate of loss through renal excretion and/or loss from the gastrointestinal tract is higher than the rate of potassium intake, potassium deficiency can occur . Therefore, maintaining a balanced diet and proper hydration can help enhance the efficacy of this compound.
Biochemical Analysis
Biochemical Properties
Potassium gluconate is involved in various biochemical reactions, primarily due to its role as a potassium source. Potassium is the most abundant intracellular cation and is essential for maintaining intracellular fluid volume and transmembrane electrochemical gradients . This compound interacts with several enzymes and proteins, including the sodium-potassium ATPase transporter, which helps maintain the electrochemical gradient across cell membranes . This interaction is crucial for proper nerve transmission, muscle contraction, and overall cellular function.
Cellular Effects
This compound influences various types of cells and cellular processes. It plays a vital role in maintaining cellular tonicity and the electrochemical gradient required for nerve transmission and muscle contraction . This compound also affects cell signaling pathways, gene expression, and cellular metabolism. For instance, it helps regulate the activity of the sodium-potassium ATPase transporter, which is essential for maintaining the resting membrane potential and cellular homeostasis .
Molecular Mechanism
The molecular mechanism of this compound involves its role as a potassium source. Potassium ions from this compound bind to the sodium-potassium ATPase transporter, facilitating the exchange of sodium and potassium ions across the cell membrane . This process is crucial for maintaining the electrochemical gradient, which is necessary for nerve transmission, muscle contraction, and other cellular functions. Additionally, this compound may influence enzyme activity and gene expression by modulating intracellular potassium levels .
Temporal Effects in Laboratory Settings
In laboratory settings, the effects of this compound can change over time. This compound is generally stable, but its long-term effects on cellular function can vary depending on the experimental conditions. Studies have shown that this compound maintains high bioavailability and absorption efficiency, with no significant differences in blood pressure or augmentation index with different doses . Long-term studies are needed to fully understand the stability, degradation, and long-term effects of this compound on cellular function.
Dosage Effects in Animal Models
The effects of this compound vary with different dosages in animal models. Studies have shown that the bioavailability of potassium from this compound is high, with absorption efficiency across all interventions being around 94% . The effects of this compound at high doses can include potential toxic or adverse effects, which need to be carefully monitored in animal studies. Threshold effects and the impact of different dosages on cellular function and overall health should be further investigated.
Metabolic Pathways
This compound is involved in several metabolic pathways, primarily through its role as a potassium source. Potassium ions from this compound interact with enzymes and cofactors involved in maintaining intracellular fluid volume and transmembrane electrochemical gradients . These interactions are crucial for proper nerve transmission, muscle contraction, and kidney function. Additionally, this compound may influence metabolic flux and metabolite levels by modulating intracellular potassium concentrations .
Transport and Distribution
This compound is transported and distributed within cells and tissues through various mechanisms. Potassium ions from this compound are absorbed via passive diffusion, primarily in the small intestine . Once absorbed, potassium is distributed throughout the body, with most of it residing intracellularly. The sodium-potassium ATPase transporter plays a key role in maintaining the intracellular concentration of potassium, which is about 30 times higher than the extracellular concentration . This distribution is essential for maintaining cellular function and overall homeostasis.
Subcellular Localization
The subcellular localization of this compound is primarily determined by the distribution of potassium ions within the cell. Potassium ions are predominantly found in the intracellular fluid, where they play a crucial role in maintaining the electrochemical gradient across cell membranes . This localization is essential for proper nerve transmission, muscle contraction, and other cellular functions. This compound may also interact with specific targeting signals or post-translational modifications that direct it to specific compartments or organelles within the cell.
Preparation Methods
Synthetic Routes and Reaction Conditions: Potassium gluconate can be synthesized by neutralizing gluconic acid with potassium hydroxide. The reaction typically involves dissolving gluconic acid in water and then adding potassium hydroxide until the solution reaches a neutral pH. The resulting solution is then evaporated to obtain this compound in crystalline form .
Industrial Production Methods: In industrial settings, this compound is produced by fermenting glucose with specific strains of bacteria to produce gluconic acid. The gluconic acid is then neutralized with potassium hydroxide to form this compound. This method ensures high purity and yield .
Chemical Reactions Analysis
Types of Reactions: Potassium gluconate primarily undergoes substitution reactions due to the presence of the gluconate ion. It can also participate in complexation reactions with metal ions.
Common Reagents and Conditions:
Substitution Reactions: this compound can react with acids to form gluconic acid and the corresponding potassium salt of the acid used.
Complexation Reactions: It can form complexes with metal ions such as calcium and magnesium under aqueous conditions.
Major Products Formed:
Substitution Reactions: Gluconic acid and potassium salts of other acids.
Complexation Reactions: Metal-gluconate complexes.
Scientific Research Applications
Potassium gluconate has a wide range of applications in scientific research:
Chemistry: It is used as a reagent in various chemical reactions and as a buffering agent in analytical chemistry.
Biology: this compound is used in cell culture media to maintain potassium levels and support cellular functions.
Medicine: It is used as a dietary supplement to treat hypokalemia (low potassium levels) and to support cardiovascular health. .
Comparison with Similar Compounds
- Potassium Bicarbonate
- Potassium Aspartate
- Potassium Phosphate
Each of these compounds has unique properties and applications, but potassium gluconate is often preferred for its palatability and non-acidifying nature.
Properties
Key on ui mechanism of action |
Potassium is the most abundant cation (approximately 150 to 160 mEq per liter) within human cells. Intracellular sodium content is relatively low. In the extracellular fluid, sodium predominates and the potassium content is low (3.5 to 5 mEq per liter). A membrane-bound enzyme, sodium-potassium–activated adenosinetriphosphatase (Na +K +ATPase), actively transports or pumps sodium out and potassium into cells to maintain the concentration gradients. The intracellular to extracellular potassium gradients are necessary for nerve impulse signaling in such specialized tissues as the heart, brain, and skeletal muscle, and for the maintenance of physiologic renal function and maintenance of acid-base balance. High intracellular potassium concentrations are necessary for numerous cellular metabolic processes. Intracellular K+ serves as a reservoir to limit the fall in extracellular potassium concentrations occurring under pathologic conditions with loss of potassium from the body. |
---|---|
CAS No. |
299-27-4 |
Molecular Formula |
C6H12KO7 |
Molecular Weight |
235.25 g/mol |
IUPAC Name |
potassium;(2R,3S,4R,5R)-2,3,4,5,6-pentahydroxyhexanoate |
InChI |
InChI=1S/C6H12O7.K/c7-1-2(8)3(9)4(10)5(11)6(12)13;/h2-5,7-11H,1H2,(H,12,13);/t2-,3-,4+,5-;/m1./s1 |
InChI Key |
RCCFGAWCGJBIIB-JJKGCWMISA-N |
SMILES |
C(C(C(C(C(C(=O)[O-])O)O)O)O)O.[K+] |
Isomeric SMILES |
C([C@H]([C@H]([C@@H]([C@H](C(=O)O)O)O)O)O)O.[K] |
Canonical SMILES |
C(C(C(C(C(C(=O)O)O)O)O)O)O.[K] |
Appearance |
Solid powder |
Color/Form |
YELLOWISH-WHITE CRYSTALS |
melting_point |
180 |
Key on ui other cas no. |
35087-77-5 299-27-4 |
physical_description |
Liquid Odourless, free flowing white to yellowish white, crystalline powder or granules Yellowish white solid; [Merck Index] Crystalline powder; [Alfa Aesar MSDS] |
Purity |
>98% (or refer to the Certificate of Analysis) |
Related CAS |
35087-77-5 |
shelf_life |
>2 years if stored properly |
solubility |
FREELY SOL IN WATER; PRACTICALLY INSOL IN ETHER, BENZENE, ABSOLUTE ALCOHOL, CHLOROFORM |
storage |
Dry, dark and at 0 - 4 C for short term (days to weeks) or -20 C for long term (months to years). |
Synonyms |
oron gluconate D-gluconate D-gluconic acid dextronic acid gluconate gluconic acid gluconic acid, (113)indium-labeled gluconic acid, (14)C-labeled gluconic acid, (159)dysprosium-labeled salt gluconic acid, (99)technecium (5+) salt gluconic acid, 1-(14)C-labeled gluconic acid, 6-(14)C-labeled gluconic acid, aluminum (3:1) salt gluconic acid, ammonium salt gluconic acid, calcium salt gluconic acid, cesium(+3) salt gluconic acid, cobalt (2:1) salt gluconic acid, copper salt gluconic acid, Fe(+2) salt, dihydrate gluconic acid, lanthanum(+3) salt gluconic acid, magnesium (2:1) salt gluconic acid, manganese (2:1) salt gluconic acid, monolithium salt gluconic acid, monopotassium salt gluconic acid, monosodium salt gluconic acid, potassium salt gluconic acid, sodium salt gluconic acid, strontium (2:1) salt gluconic acid, tin(+2) salt gluconic acid, zinc salt lithium gluconate magnerot magnesium gluconate maltonic acid manganese gluconate pentahydroxycaproic acid sodium gluconate zinc gluconate |
Origin of Product |
United States |
Retrosynthesis Analysis
AI-Powered Synthesis Planning: Our tool employs the Template_relevance Pistachio, Template_relevance Bkms_metabolic, Template_relevance Pistachio_ringbreaker, Template_relevance Reaxys, Template_relevance Reaxys_biocatalysis model, leveraging a vast database of chemical reactions to predict feasible synthetic routes.
One-Step Synthesis Focus: Specifically designed for one-step synthesis, it provides concise and direct routes for your target compounds, streamlining the synthesis process.
Accurate Predictions: Utilizing the extensive PISTACHIO, BKMS_METABOLIC, PISTACHIO_RINGBREAKER, REAXYS, REAXYS_BIOCATALYSIS database, our tool offers high-accuracy predictions, reflecting the latest in chemical research and data.
Strategy Settings
Precursor scoring | Relevance Heuristic |
---|---|
Min. plausibility | 0.01 |
Model | Template_relevance |
Template Set | Pistachio/Bkms_metabolic/Pistachio_ringbreaker/Reaxys/Reaxys_biocatalysis |
Top-N result to add to graph | 6 |
Feasible Synthetic Routes
Q1: Does potassium gluconate directly interact with specific cellular targets, or are its effects primarily mediated through changes in potassium levels?
A1: While this compound can contribute to changes in potassium levels, research suggests potential direct interactions with cellular processes. Studies using intestinal brush border membrane vesicles (BBMVs) have shown that this compound can directly affect active sodium/glucose uptake, possibly by competing with sodium for binding sites on the sodium/glucose cotransporter [, ].
Q2: What is the molecular formula and weight of this compound?
A2: this compound has a molecular formula of C6H11KO7 and a molecular weight of 234.25 g/mol.
Q3: Is there any available spectroscopic data (e.g., IR, NMR) characterizing this compound?
A3: Yes, spectroscopic data is available. For example, one study used IR and 1H NMR to confirm the structure of D-glucose acid-δ-lactone synthesized from this compound [].
Q4: Has this compound been investigated for use in anti-icing formulations, and if so, what are the findings regarding its performance and material compatibility?
A4: Research indicates this compound exhibits promise as a component in anti-icing formulations. A study exploring locally sourced brine additives found that formulations containing this compound demonstrated favorable ice-melting properties and significantly reduced corrosion rates on carbon steel compared to traditional deicers like sodium chloride and magnesium chloride [].
Q5: Does this compound exhibit any catalytic properties?
A5: There is no direct evidence within the provided research suggesting inherent catalytic properties of this compound itself.
Q6: Have there been any computational studies investigating this compound?
A6: The provided research doesn't delve into computational studies specifically on this compound.
Q7: How does modifying the structure of this compound, such as changing the potassium cation to another metal, affect its activity or properties?
A7: While specific SAR studies on this compound weren't described in the provided research, substituting potassium with other metals can significantly impact the compound's properties. For instance, one study synthesized various metal gluconates like calcium gluconate, sodium gluconate, and zinc gluconate, each possessing distinct characteristics and applications [].
Q8: How does this compound perform in different formulations, particularly regarding its stability and bioavailability?
A8: Research suggests that this compound demonstrates good stability and bioavailability in various formulations. One study comparing the bioavailability of potassium from potatoes and this compound supplements found no significant difference in serum potassium AUC, indicating comparable absorption efficiency from both sources [, ]. Another study explored the suitability of different this compound dosage forms (powder, granules, pulverized tablets) for administration to newborns via feeding tubes, highlighting the importance of formulation on drug delivery [].
Q9: What is the impact of this compound on potassium levels in the body, and how is it absorbed, distributed, metabolized, and excreted?
A9: this compound serves as a source of potassium, an essential electrolyte. While the studies don't provide a detailed ADME profile, they highlight its effectiveness in treating hypokalemia []. A study on cats with chronic kidney disease demonstrated that oral this compound effectively increased serum potassium levels, confirming its absorption and systemic distribution []. Another study in humans showed that the bioavailability of potassium from potatoes and this compound supplements was comparable, implying similar absorption and utilization [].
Q10: What are the major findings from in vitro and in vivo studies evaluating the effects of this compound?
A10: In vitro studies using rat erythrocytes showed that this compound increased active potassium transport, potentially by influencing glycolysis []. In vivo studies in cats with chronic kidney disease demonstrated that this compound effectively treated hypokalemia, highlighting its therapeutic potential in managing electrolyte imbalances []. Additionally, research on human subjects compared the bioavailability of potassium from potatoes and this compound supplements, finding no significant difference in serum potassium levels, suggesting comparable efficacy in delivering potassium [].
Disclaimer and Information on In-Vitro Research Products
Please be aware that all articles and product information presented on BenchChem are intended solely for informational purposes. The products available for purchase on BenchChem are specifically designed for in-vitro studies, which are conducted outside of living organisms. In-vitro studies, derived from the Latin term "in glass," involve experiments performed in controlled laboratory settings using cells or tissues. It is important to note that these products are not categorized as medicines or drugs, and they have not received approval from the FDA for the prevention, treatment, or cure of any medical condition, ailment, or disease. We must emphasize that any form of bodily introduction of these products into humans or animals is strictly prohibited by law. It is essential to adhere to these guidelines to ensure compliance with legal and ethical standards in research and experimentation.