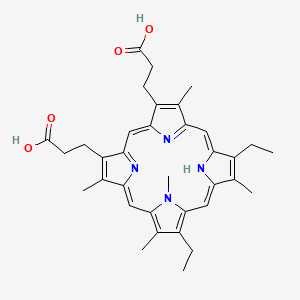
N-methyl mesoporphyrin IX
Overview
Description
N-methyl Mesoporphyrin IX is a water-soluble, non-symmetric porphyrin with excellent optical properties and exceptional selectivity for G-quadruplex DNA. G-quadruplexes are non-canonical DNA structures formed by guanine-rich sequences, which are implicated in genomic stability, longevity, and cancer . The ability of this compound to selectively recognize G-quadruplex structures makes it a valuable scaffold for designing novel G-quadruplex binders .
Mechanism of Action
Target of Action
N-Methyl Mesoporphyrin IX (NMM) is a water-soluble, non-symmetric porphyrin . Its primary target is G-quadruplex (GQ) DNA , which are non-canonical DNA structures formed by guanine-rich sequences . GQs are implicated in genomic stability, longevity, and cancer .
Mode of Action
NMM has unparalleled selectivity for GQ DNA . It selectively recognizes GQ structures , making it a valuable scaffold for designing novel GQ binders . The ability of NMM to selectively recognize GQ structures is due to its excellent optical properties .
Biochemical Pathways
The interaction of NMM with GQ DNA affects the stability of the GQ structures . NMM has the ability to stabilize GQ structures and favor parallel GQ conformations . This interaction plays a role in genomic stability, longevity, and cancer .
Pharmacokinetics
It is known that nmm is water-soluble , which could potentially influence its absorption and distribution in the body.
Result of Action
The binding of NMM to GQ DNA induces high fluorescence . This “light-switch effect” is a result of the interaction between NMM and GQ DNA . This property of NMM has been utilized in the development of biosensors for heavy metals, small molecules (e.g., ATP and pesticides), DNA, and proteins .
Action Environment
The action of NMM is influenced by environmental factors. For instance, NMM is sensitive to G-quadruplex DNA but unreactive to duplex, triplex, and single-stranded forms of DNA . Furthermore, NMM is stable in a cool, dry environment but can degrade when exposed to strong light, heat, acid, and strong oxidizers .
Biochemical Analysis
Biochemical Properties
N-methyl mesoporphyrin IX has the ability to selectively recognize GQ structures . This makes it a valuable scaffold for designing novel GQ binders . It is also an inhibitor of ferrochelatase , an enzyme involved in heme synthesis .
Cellular Effects
This compound is an in situ inhibitor and an ex situ monitor for Aβ amyloidogenesis both in vitro and in cells . It is sensitive to G-quadruplexes DNA but has no response to duplexes, triplexes and single-stranded forms DNA .
Molecular Mechanism
Upon binding to quadruplex DNA, this compound can adjust its macrocycle geometry to match the terminal face of a G-quadruplex, leading to an enhancement in its fluorescence .
Temporal Effects in Laboratory Settings
This compound is stable in cold, dry environments . It degrades when exposed to strong light, heat, acid, and strong oxidants .
Metabolic Pathways
This compound is a transition state analog of porphyrin and an inhibitor of ferrochelatase . It inhibits ferrochelatase in mouse liver mitochondria in vitro and in vivo, leading to an accumulation of porphyrin in isolated liver mitochondria .
Preparation Methods
Synthetic Routes and Reaction Conditions: N-methyl Mesoporphyrin IX can be synthesized through a series of chemical reactions involving the methylation of mesoporphyrin IX. The synthesis typically involves the use of methylating agents such as methyl iodide or dimethyl sulfate under controlled conditions .
Industrial Production Methods: Industrial production of this compound involves large-scale synthesis using optimized reaction conditions to ensure high yield and purity. The process includes purification steps such as chromatography to isolate the desired compound .
Chemical Reactions Analysis
Types of Reactions: N-methyl Mesoporphyrin IX undergoes various chemical reactions, including:
Oxidation: The compound can be oxidized under specific conditions to form oxidized derivatives.
Reduction: Reduction reactions can convert this compound into reduced forms.
Substitution: Substitution reactions can introduce different functional groups into the porphyrin ring.
Common Reagents and Conditions:
Oxidation: Common oxidizing agents include hydrogen peroxide and potassium permanganate.
Reduction: Reducing agents such as sodium borohydride are used.
Substitution: Reagents like halogens and alkylating agents are employed.
Major Products: The major products formed from these reactions include various derivatives of this compound with altered functional groups and oxidation states .
Scientific Research Applications
N-methyl Mesoporphyrin IX has a wide range of scientific research applications, including:
Comparison with Similar Compounds
Mesoporphyrin IX: The unmethylated analogue of N-methyl Mesoporphyrin IX, which lacks the ability to stabilize G-quadruplex DNA.
Protoporphyrin IX: Another porphyrin compound with different binding properties and applications.
Uniqueness: this compound is unique due to its exceptional selectivity for G-quadruplex DNA and its ability to induce high fluorescence upon binding. This makes it a valuable tool for studying G-quadruplex structures and their roles in biological processes .
Properties
IUPAC Name |
3-[18-(2-carboxyethyl)-7,12-diethyl-3,8,13,17,22-pentamethyl-23H-porphyrin-2-yl]propanoic acid | |
---|---|---|
Source | PubChem | |
URL | https://pubchem.ncbi.nlm.nih.gov | |
Description | Data deposited in or computed by PubChem | |
InChI |
InChI=1S/C35H40N4O4/c1-8-22-18(3)26-14-27-19(4)24(10-12-34(40)41)29(36-27)15-30-25(11-13-35(42)43)20(5)28(38-30)16-33-23(9-2)21(6)32(39(33)7)17-31(22)37-26/h14-17,37H,8-13H2,1-7H3,(H,40,41)(H,42,43) | |
Source | PubChem | |
URL | https://pubchem.ncbi.nlm.nih.gov | |
Description | Data deposited in or computed by PubChem | |
InChI Key |
XODZICXILWCPBD-UHFFFAOYSA-N | |
Source | PubChem | |
URL | https://pubchem.ncbi.nlm.nih.gov | |
Description | Data deposited in or computed by PubChem | |
Canonical SMILES |
CCC1=C(C2=CC3=NC(=CC4=NC(=CC5=C(C(=C(N5C)C=C1N2)C)CC)C(=C4CCC(=O)O)C)C(=C3C)CCC(=O)O)C | |
Source | PubChem | |
URL | https://pubchem.ncbi.nlm.nih.gov | |
Description | Data deposited in or computed by PubChem | |
Molecular Formula |
C35H40N4O4 | |
Source | PubChem | |
URL | https://pubchem.ncbi.nlm.nih.gov | |
Description | Data deposited in or computed by PubChem | |
DSSTOX Substance ID |
DTXSID70349630 | |
Record name | N-methyl mesoporphyrin IX | |
Source | EPA DSSTox | |
URL | https://comptox.epa.gov/dashboard/DTXSID70349630 | |
Description | DSSTox provides a high quality public chemistry resource for supporting improved predictive toxicology. | |
Molecular Weight |
580.7 g/mol | |
Source | PubChem | |
URL | https://pubchem.ncbi.nlm.nih.gov | |
Description | Data deposited in or computed by PubChem | |
CAS No. |
142234-85-3 | |
Record name | N-methyl mesoporphyrin IX | |
Source | EPA DSSTox | |
URL | https://comptox.epa.gov/dashboard/DTXSID70349630 | |
Description | DSSTox provides a high quality public chemistry resource for supporting improved predictive toxicology. | |
Record name | 21H,23H-porphine-2,18-dipropanoic acid, 8,13-diethyl-3,7,12,17,23-pentamethyl | |
Source | European Chemicals Agency (ECHA) | |
URL | https://echa.europa.eu/information-on-chemicals | |
Description | The European Chemicals Agency (ECHA) is an agency of the European Union which is the driving force among regulatory authorities in implementing the EU's groundbreaking chemicals legislation for the benefit of human health and the environment as well as for innovation and competitiveness. | |
Explanation | Use of the information, documents and data from the ECHA website is subject to the terms and conditions of this Legal Notice, and subject to other binding limitations provided for under applicable law, the information, documents and data made available on the ECHA website may be reproduced, distributed and/or used, totally or in part, for non-commercial purposes provided that ECHA is acknowledged as the source: "Source: European Chemicals Agency, http://echa.europa.eu/". Such acknowledgement must be included in each copy of the material. ECHA permits and encourages organisations and individuals to create links to the ECHA website under the following cumulative conditions: Links can only be made to webpages that provide a link to the Legal Notice page. | |
Retrosynthesis Analysis
AI-Powered Synthesis Planning: Our tool employs the Template_relevance Pistachio, Template_relevance Bkms_metabolic, Template_relevance Pistachio_ringbreaker, Template_relevance Reaxys, Template_relevance Reaxys_biocatalysis model, leveraging a vast database of chemical reactions to predict feasible synthetic routes.
One-Step Synthesis Focus: Specifically designed for one-step synthesis, it provides concise and direct routes for your target compounds, streamlining the synthesis process.
Accurate Predictions: Utilizing the extensive PISTACHIO, BKMS_METABOLIC, PISTACHIO_RINGBREAKER, REAXYS, REAXYS_BIOCATALYSIS database, our tool offers high-accuracy predictions, reflecting the latest in chemical research and data.
Strategy Settings
Precursor scoring | Relevance Heuristic |
---|---|
Min. plausibility | 0.01 |
Model | Template_relevance |
Template Set | Pistachio/Bkms_metabolic/Pistachio_ringbreaker/Reaxys/Reaxys_biocatalysis |
Top-N result to add to graph | 6 |
Feasible Synthetic Routes
Q1: What is the primary biological target of NMM?
A1: NMM exhibits exceptional selectivity for G-quadruplex (GQ) DNA over duplex DNA. [, , ] GQs are non-canonical DNA structures formed by guanine-rich sequences found in telomeres and gene promoters. []
Q2: How does NMM interact with G-quadruplex DNA?
A2: NMM interacts with GQs through end-stacking interactions, primarily with the terminal G-tetrad. [, ] Its N-methyl group plays a crucial role in this selectivity by fitting into the GQ core and aligning with potassium ions, while causing steric clashes with duplex DNA or antiparallel GQs. []
Q3: What are the downstream effects of NMM binding to GQs?
A3: NMM binding stabilizes the GQ structure, often favoring the parallel conformation over antiparallel or hybrid forms. [, , ] This stabilization can influence biological processes like telomere maintenance, gene expression, and DNA replication. [, , ]
Q4: Does NMM affect heme synthesis?
A4: Yes, in certain organisms like Euglena gracilis and Cyanidium caldarium, NMM inhibits heme synthesis by blocking iron insertion into protoporphyrin IX. [, ] This inhibition leads to increased delta-aminolevulinic acid synthase activity and protoporphyrin IX accumulation. [, ]
Q5: What is the molecular formula and weight of NMM?
A5: The molecular formula of NMM is C35H38N4O4, and its molecular weight is 578.7 g/mol.
Q6: What are the key spectroscopic characteristics of NMM?
A6: NMM exhibits distinct spectroscopic properties:
- UV-Vis Spectroscopy: NMM shows characteristic absorption bands in the UV-Vis region, which are sensitive to its environment and can be used to study its interactions with GQs. [, ]
- Fluorescence Spectroscopy: NMM exhibits a "light-switch" effect, meaning its fluorescence intensity dramatically increases upon binding to GQs. [] This property makes it a valuable probe for studying GQ formation and dynamics. [, , ]
Q7: Does NMM possess any catalytic properties?
A7: While not a catalyst itself, NMM can enhance the catalytic activity of certain systems:
- DNAzyme Activity: NMM binding to specific G-quadruplexes can significantly enhance their peroxidase-like activity. [, ] This property makes NMM-GQ complexes promising candidates for developing biosensors and catalytic tools. [, , , , , ]
Q8: How crucial is the N-methyl group for NMM's activity and selectivity?
A8: The N-methyl group is essential for NMM's selectivity towards GQs. [, ] Mesoporphyrin IX, lacking the N-methyl group, does not exhibit the same stabilizing effect on GQs. [] This difference highlights the importance of the N-methyl group for specific interactions with the GQ structure.
Q9: What in vitro applications have been explored for NMM?
A9: NMM's GQ-binding properties have led to its use in various in vitro applications, including:
- Fluorescent probe for GQs: NMM's "light-switch" effect makes it a valuable tool for studying GQ formation, stability, and interactions with other molecules. [, , , , , , , , , , , ]
- Biosensor development: NMM-GQ complexes have been used to develop sensitive and selective biosensors for detecting various analytes, including metal ions, small molecules, and enzymes. [, , , , , , , , ]
Q10: Are there any in vivo studies demonstrating NMM's efficacy?
A10: A study on tobacco mosaic virus (TMV) showed that NMM, by stabilizing a G4 structure in the TMV genome, inhibited viral proliferation in tobacco plants. [] This finding highlights NMM's potential as an antiviral agent.
Disclaimer and Information on In-Vitro Research Products
Please be aware that all articles and product information presented on BenchChem are intended solely for informational purposes. The products available for purchase on BenchChem are specifically designed for in-vitro studies, which are conducted outside of living organisms. In-vitro studies, derived from the Latin term "in glass," involve experiments performed in controlled laboratory settings using cells or tissues. It is important to note that these products are not categorized as medicines or drugs, and they have not received approval from the FDA for the prevention, treatment, or cure of any medical condition, ailment, or disease. We must emphasize that any form of bodily introduction of these products into humans or animals is strictly prohibited by law. It is essential to adhere to these guidelines to ensure compliance with legal and ethical standards in research and experimentation.