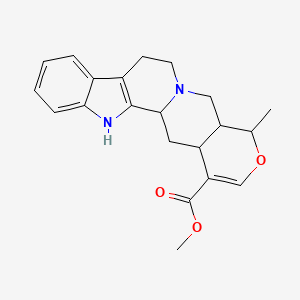
Ajmalicine
Overview
Description
Mechanism of Action
Target of Action
Ajmalicine primarily targets the α1-adrenergic receptors, where it acts as an antagonist . It also has a strong inhibitory effect on the CYP2D6 liver enzyme . In the context of Alzheimer’s disease, this compound has been identified as a multi-target directed ligand, affecting several disease-modifying targets such as amyloid beta, beta-secretase, monoaminoxidase-B, and cholinesterase .
Mode of Action
This compound interacts with its targets by changing the shape and threshold of cardiac action potentials . It produces potent sodium channel blocking effects , which makes it a very useful drug for acute intravenous treatments . In Alzheimer’s disease, this compound inhibits the activity of enzymes such as cholinesterase, β-site amyloid cleaving enzyme (BACE-1), and monoamine oxidase-B (MAO-B) .
Biochemical Pathways
This compound affects the terpenoid indole alkaloid (TIA) pathway . The TIA pathway involves several genes, including G10H, 10HGO, TDC, SLS, STR, and SDG . The STR gene, encoding strictosidine synthase, has been identified as a regulatory gene in the this compound biosynthesis .
Pharmacokinetics
It is known that this compound has a very short half-life, which contributes to its usefulness in acute intravenous treatments .
Result of Action
This compound’s interaction with its targets leads to various molecular and cellular effects. It has been shown to have neuroprotective activity against Aβ42 and H2O2 induced toxicity in PC12 cells . Additionally, this compound has been found to induce pyroptosis, a form of programmed cell death, in hepatoma cells .
Action Environment
Environmental factors can influence the action, efficacy, and stability of this compound. For instance, the use of silver nanoparticles as elicitors in cell cultures has been shown to increase the production of this compound . Similarly, stress factors such as mannitol, sodium chloride, potassium chloride, cadmium chloride, and PVP polyvinyl pyrrolidone K-30 have been shown to enhance this compound accumulation under in-vitro conditions .
Biochemical Analysis
Biochemical Properties
Ajmalicine plays a significant role in various biochemical reactions. It acts as an α1-adrenergic receptor antagonist with preferential actions over α2-adrenergic receptors, which underlies its hypotensive effects . This compound interacts with several enzymes and proteins, including strictosidine synthase and strictosidine glucosidase, which are crucial in its biosynthesis . The compound also inhibits the CYP2D6 liver enzyme, affecting the metabolism of various drugs .
Cellular Effects
This compound influences several cellular processes. It has been shown to increase cerebral blood flow, which can improve oxygen bioavailability and potentially aid in the treatment of Alzheimer’s disease . This compound also exhibits cytotoxic effects on certain cancer cell lines and has been observed to modulate cell signaling pathways, gene expression, and cellular metabolism .
Molecular Mechanism
At the molecular level, this compound exerts its effects by binding to α1-adrenergic receptors, thereby inhibiting their activity . This interaction leads to vasodilation and reduced blood pressure. This compound also inhibits the CYP2D6 enzyme, which plays a role in drug metabolism . Additionally, it has been shown to interact with strictosidine synthase and strictosidine glucosidase during its biosynthesis .
Temporal Effects in Laboratory Settings
In laboratory settings, the effects of this compound have been studied over time using genetically encoded Fluorescent Resonance Energy Transfer (FRET)-based nanosensors . These studies have shown that this compound levels can be monitored with high spatial and temporal resolution, providing insights into its stability and degradation . Long-term studies have indicated that this compound maintains its efficacy over extended periods, with minimal degradation .
Dosage Effects in Animal Models
The effects of this compound vary with different dosages in animal models. At therapeutic doses, this compound effectively reduces blood pressure without significant adverse effects . At higher doses, it can exhibit toxic effects, including central nervous system depression and potential hepatotoxicity . Threshold effects have been observed, indicating that there is a narrow therapeutic window for this compound .
Metabolic Pathways
This compound is involved in the terpenoid indole alkaloid (TIA) pathway . The biosynthesis of this compound involves the terpenoid moiety synthesized by the MEP pathway and the indole moiety synthesized by the indole pathway . Key enzymes involved in its biosynthesis include strictosidine synthase and strictosidine glucosidase . This compound’s metabolic flux can be monitored using FRET-based nanosensors, providing insights into its regulation .
Transport and Distribution
This compound is transported and distributed within cells and tissues through passive diffusion and active transport mechanisms . It is accumulated within vacuoles against a concentration gradient, primarily due to the relative acidity of the vacuolar compartment . This dynamic distribution is influenced by the pH difference between the vacuole and the medium .
Subcellular Localization
The subcellular localization of this compound involves its accumulation in vacuoles . The enzymes involved in its biosynthesis, such as strictosidine synthase, are also localized in specific subcellular compartments . This localization is crucial for the efficient biosynthesis and storage of this compound within plant cells .
Preparation Methods
Synthetic Routes and Reaction Conditions: Raubasine can be synthesized through the biosynthesis of its precursor, strictosidine. The process involves the terpenoid and indole moieties. The terpenoid moiety is synthesized via the MEP pathway, starting with pyruvate and D-glyceraldehyde-3-phosphate. The indole moiety is synthesized from tryptophan, catalyzed by tryptophan decarboxylase to form tryptamine. Strictosidine synthase then catalyzes the formation of strictosidine, which is the common precursor for all terpenoid indole alkaloids .
Industrial Production Methods: Industrial production of raubasine involves extraction from natural sources such as Rauvolfia species and Catharanthus roseus. The extraction process typically includes solvent extraction, purification, and crystallization to obtain the pure compound .
Chemical Reactions Analysis
Types of Reactions: Raubasine undergoes various chemical reactions, including:
Oxidation: Raubasine can be oxidized to form different derivatives.
Reduction: It can be reduced to form dihydro derivatives.
Substitution: Raubasine can undergo substitution reactions, particularly at the indole nitrogen.
Common Reagents and Conditions:
Oxidation: Common oxidizing agents include potassium permanganate and hydrogen peroxide.
Reduction: Reducing agents such as sodium borohydride and lithium aluminum hydride are used.
Substitution: Substitution reactions often involve reagents like alkyl halides and acyl chlorides.
Major Products: The major products formed from these reactions include various derivatives of raubasine, such as dihydroajmalicine and other substituted indole alkaloids .
Scientific Research Applications
Raubasine has a wide range of scientific research applications:
Chemistry: It is used as a precursor for synthesizing other indole alkaloids.
Biology: Raubasine is studied for its role in plant metabolism and biosynthesis pathways.
Industry: Raubasine is used in the pharmaceutical industry for the production of antihypertensive drugs
Comparison with Similar Compounds
Yohimbine: Another indole alkaloid with similar structure but different pharmacological effects.
Rauwolscine: Structurally related to raubasine but acts as an alpha2-adrenoceptor antagonist.
Corynanthine: Similar in structure but has different receptor binding affinities.
Uniqueness: Raubasine is unique in its selective action on alpha1-adrenergic receptors, which distinguishes it from other similar compounds that may act on different receptors or have different pharmacological profiles .
Properties
CAS No. |
483-04-5 |
---|---|
Molecular Formula |
C21H24N2O3 |
Molecular Weight |
352.4 g/mol |
IUPAC Name |
methyl (15S,16S,20S)-16-methyl-17-oxa-3,13-diazapentacyclo[11.8.0.02,10.04,9.015,20]henicosa-2(10),4,6,8,18-pentaene-19-carboxylate |
InChI |
InChI=1S/C21H24N2O3/c1-12-16-10-23-8-7-14-13-5-3-4-6-18(13)22-20(14)19(23)9-15(16)17(11-26-12)21(24)25-2/h3-6,11-12,15-16,19,22H,7-10H2,1-2H3/t12-,15-,16-,19?/m0/s1 |
InChI Key |
GRTOGORTSDXSFK-WADGIJBFSA-N |
SMILES |
CC1C2CN3CCC4=C(C3CC2C(=CO1)C(=O)OC)NC5=CC=CC=C45 |
Isomeric SMILES |
C[C@H]1[C@@H]2CN3CCC4=C(C3C[C@@H]2C(=CO1)C(=O)OC)NC5=CC=CC=C45 |
Canonical SMILES |
CC1C2CN3CCC4=C(C3CC2C(=CO1)C(=O)OC)NC5=CC=CC=C45 |
Appearance |
Solid powder |
Key on ui other cas no. |
483-04-5 6474-90-4 |
Pictograms |
Irritant |
Purity |
>98% (or refer to the Certificate of Analysis) |
shelf_life |
>3 years if stored properly |
solubility |
Soluble in DMSO |
storage |
Dry, dark and at 0 - 4 C for short term (days to weeks) or -20 C for long term (months to years). |
Synonyms |
19-epiajmalicine ajmalicine ajmalicine hydrochloride ajmalicine, (19alpha,20alpha)-isomer ajmalicine, (19beta)-isomer ajmalicine, (19beta,20alpha)-isomer ajmalicine, (3-beta,19beta)-isomer ajmalicine, (3beta,19alpha)-isomer ajmalicine, (3beta,19alpha,20alpha)-isomer ajmalicine, (hydrochloride(19beta,20alpha))-isomer ajmalicine, hydrochloride(19alpha)-isomer ajmalicine, PO4(19alpha)-isomer akuammigine delta-yohimbine Lamuran raubasine raubasine HCl raubasine hydrochloride rauvasan tetrahydro-alstonine tetrahydroalstonine |
Origin of Product |
United States |
Retrosynthesis Analysis
AI-Powered Synthesis Planning: Our tool employs the Template_relevance Pistachio, Template_relevance Bkms_metabolic, Template_relevance Pistachio_ringbreaker, Template_relevance Reaxys, Template_relevance Reaxys_biocatalysis model, leveraging a vast database of chemical reactions to predict feasible synthetic routes.
One-Step Synthesis Focus: Specifically designed for one-step synthesis, it provides concise and direct routes for your target compounds, streamlining the synthesis process.
Accurate Predictions: Utilizing the extensive PISTACHIO, BKMS_METABOLIC, PISTACHIO_RINGBREAKER, REAXYS, REAXYS_BIOCATALYSIS database, our tool offers high-accuracy predictions, reflecting the latest in chemical research and data.
Strategy Settings
Precursor scoring | Relevance Heuristic |
---|---|
Min. plausibility | 0.01 |
Model | Template_relevance |
Template Set | Pistachio/Bkms_metabolic/Pistachio_ringbreaker/Reaxys/Reaxys_biocatalysis |
Top-N result to add to graph | 6 |
Feasible Synthetic Routes
Disclaimer and Information on In-Vitro Research Products
Please be aware that all articles and product information presented on BenchChem are intended solely for informational purposes. The products available for purchase on BenchChem are specifically designed for in-vitro studies, which are conducted outside of living organisms. In-vitro studies, derived from the Latin term "in glass," involve experiments performed in controlled laboratory settings using cells or tissues. It is important to note that these products are not categorized as medicines or drugs, and they have not received approval from the FDA for the prevention, treatment, or cure of any medical condition, ailment, or disease. We must emphasize that any form of bodily introduction of these products into humans or animals is strictly prohibited by law. It is essential to adhere to these guidelines to ensure compliance with legal and ethical standards in research and experimentation.