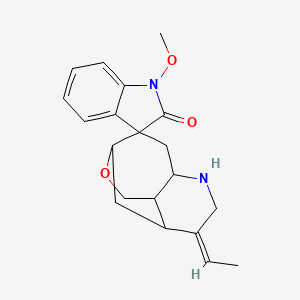
Rankinidine
Overview
Description
Rankinidine is an oxindole alkaloid isolated from the methanol extract of the stem of Gelsemium rankinii. It has a molecular formula of C20H24N2O3 and a molecular weight of 340.42 g/mol
Preparation Methods
Synthetic Routes and Reaction Conditions: The synthesis of Rankinidine involves several steps, starting from readily available precursors. One common method includes the cyclization of an appropriate precursor under specific conditions to form the oxindole core. The reaction conditions typically involve the use of strong acids or bases, high temperatures, and sometimes the presence of catalysts to facilitate the cyclization process .
Industrial Production Methods: Industrial production of this compound may involve large-scale synthesis using optimized reaction conditions to ensure high yield and purity. The process often includes purification steps such as recrystallization or chromatography to isolate the desired compound from by-products and impurities .
Chemical Reactions Analysis
Types of Reactions: Rankinidine undergoes various chemical reactions, including:
Oxidation: this compound can be oxidized to form different derivatives, depending on the oxidizing agent and conditions used.
Reduction: Reduction reactions can modify the functional groups present in this compound, leading to the formation of reduced derivatives.
Substitution: this compound can undergo substitution reactions where specific atoms or groups in the molecule are replaced by other atoms or groups.
Common Reagents and Conditions:
Oxidation: Common oxidizing agents include potassium permanganate and chromium trioxide.
Reduction: Reducing agents such as lithium aluminum hydride or sodium borohydride are often used.
Substitution: Various reagents, including halogens and nucleophiles, can be used under appropriate conditions to achieve substitution reactions.
Major Products: The major products formed from these reactions depend on the specific reagents and conditions used. For example, oxidation may yield oxindole derivatives, while reduction can produce reduced forms of this compound .
Scientific Research Applications
Rankinidine has several applications in scientific research, including:
Chemistry: Used as a precursor for synthesizing other complex molecules and studying reaction mechanisms.
Biology: Investigated for its potential biological activities, including antimicrobial and anticancer properties.
Medicine: Explored for its therapeutic potential in treating various diseases due to its unique chemical structure.
Industry: Utilized in the development of new materials and chemical processes
Mechanism of Action
The mechanism of action of Rankinidine involves its interaction with specific molecular targets and pathways. It is believed to exert its effects by binding to certain receptors or enzymes, thereby modulating their activity. This interaction can lead to various biological responses, such as inhibition of cell proliferation or induction of apoptosis in cancer cells .
Comparison with Similar Compounds
Ranitidine: A histamine H2 receptor antagonist used to reduce stomach acid production.
Famotidine: Another histamine H2 receptor antagonist with similar applications as Ranitidine.
Cimetidine: A histamine H2 receptor antagonist used to treat gastric acid-related disorders.
Uniqueness of Rankinidine: this compound is unique due to its oxindole alkaloid structure, which distinguishes it from other histamine H2 receptor antagonists.
Properties
IUPAC Name |
(7Z)-7-ethylidene-1'-methoxyspiro[11-oxa-5-azatricyclo[6.3.1.04,9]dodecane-2,3'-indole]-2'-one | |
---|---|---|
Source | PubChem | |
URL | https://pubchem.ncbi.nlm.nih.gov | |
Description | Data deposited in or computed by PubChem | |
InChI |
InChI=1S/C20H24N2O3/c1-3-12-10-21-16-9-20(18-8-13(12)14(16)11-25-18)15-6-4-5-7-17(15)22(24-2)19(20)23/h3-7,13-14,16,18,21H,8-11H2,1-2H3/b12-3+ | |
Source | PubChem | |
URL | https://pubchem.ncbi.nlm.nih.gov | |
Description | Data deposited in or computed by PubChem | |
InChI Key |
ZXRGGMATGWCUBP-KGVSQERTSA-N | |
Source | PubChem | |
URL | https://pubchem.ncbi.nlm.nih.gov | |
Description | Data deposited in or computed by PubChem | |
Canonical SMILES |
CC=C1CNC2CC3(C4CC1C2CO4)C5=CC=CC=C5N(C3=O)OC | |
Source | PubChem | |
URL | https://pubchem.ncbi.nlm.nih.gov | |
Description | Data deposited in or computed by PubChem | |
Isomeric SMILES |
C/C=C/1\CNC2CC3(C4CC1C2CO4)C5=CC=CC=C5N(C3=O)OC | |
Source | PubChem | |
URL | https://pubchem.ncbi.nlm.nih.gov | |
Description | Data deposited in or computed by PubChem | |
Molecular Formula |
C20H24N2O3 | |
Source | PubChem | |
URL | https://pubchem.ncbi.nlm.nih.gov | |
Description | Data deposited in or computed by PubChem | |
Molecular Weight |
340.4 g/mol | |
Source | PubChem | |
URL | https://pubchem.ncbi.nlm.nih.gov | |
Description | Data deposited in or computed by PubChem | |
CAS No. |
106466-66-4 | |
Record name | Rankinidine | |
Source | ChemIDplus | |
URL | https://pubchem.ncbi.nlm.nih.gov/substance/?source=chemidplus&sourceid=0106466664 | |
Description | ChemIDplus is a free, web search system that provides access to the structure and nomenclature authority files used for the identification of chemical substances cited in National Library of Medicine (NLM) databases, including the TOXNET system. | |
Retrosynthesis Analysis
AI-Powered Synthesis Planning: Our tool employs the Template_relevance Pistachio, Template_relevance Bkms_metabolic, Template_relevance Pistachio_ringbreaker, Template_relevance Reaxys, Template_relevance Reaxys_biocatalysis model, leveraging a vast database of chemical reactions to predict feasible synthetic routes.
One-Step Synthesis Focus: Specifically designed for one-step synthesis, it provides concise and direct routes for your target compounds, streamlining the synthesis process.
Accurate Predictions: Utilizing the extensive PISTACHIO, BKMS_METABOLIC, PISTACHIO_RINGBREAKER, REAXYS, REAXYS_BIOCATALYSIS database, our tool offers high-accuracy predictions, reflecting the latest in chemical research and data.
Strategy Settings
Precursor scoring | Relevance Heuristic |
---|---|
Min. plausibility | 0.01 |
Model | Template_relevance |
Template Set | Pistachio/Bkms_metabolic/Pistachio_ringbreaker/Reaxys/Reaxys_biocatalysis |
Top-N result to add to graph | 6 |
Feasible Synthetic Routes
Q1: What is Rankinidine and where is it found?
A1: this compound is an oxindole alkaloid found in the Gelsemium genus, specifically isolated from the stem of Gelsemium rankinii []. It belongs to the humantenine-type alkaloids, which are known for their structural complexity and potential biological activity [, ].
Q2: How is this compound metabolized in different species?
A2: Research using high-performance liquid chromatography/quadrupole time-of-flight mass spectrometry (LC-QTOF) identified 11 metabolites of this compound and five main metabolic pathways: demethylation, reduction, oxidation, oxidation & reduction, and demethylation & oxidation []. Significantly, there are qualitative and quantitative species-specific differences in this compound metabolism. For example, pigs and goats showed a higher capacity for this compound oxidation compared to humans and rats, while the latter two exhibited higher demethylation and reduction abilities [].
Q3: What are the implications of these metabolic differences?
A3: The species-specific metabolic differences of this compound in human, pig, goat, and rat liver microsomes could help explain the variation in toxicity observed with Gelsemium species []. Understanding these metabolic pathways is crucial for future in vivo studies and for assessing the potential risks and benefits of this compound and related compounds.
Q4: Has the synthesis of this compound been explored?
A4: Yes, researchers have investigated copper(II)-mediated oxidative coupling reactions as a route to synthesize nitrogen-heterocycles, including the oxindole core structure found in this compound []. This approach holds promise for developing efficient synthetic strategies for this compound and other structurally complex alkaloids.
Disclaimer and Information on In-Vitro Research Products
Please be aware that all articles and product information presented on BenchChem are intended solely for informational purposes. The products available for purchase on BenchChem are specifically designed for in-vitro studies, which are conducted outside of living organisms. In-vitro studies, derived from the Latin term "in glass," involve experiments performed in controlled laboratory settings using cells or tissues. It is important to note that these products are not categorized as medicines or drugs, and they have not received approval from the FDA for the prevention, treatment, or cure of any medical condition, ailment, or disease. We must emphasize that any form of bodily introduction of these products into humans or animals is strictly prohibited by law. It is essential to adhere to these guidelines to ensure compliance with legal and ethical standards in research and experimentation.