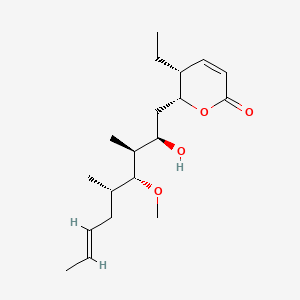
Pironetin
Overview
Description
Pironetin is a bacterial metabolite originally isolated from Streptomyces that has diverse biological activities, including anti-proliferative, immunosuppressive, and plant growth regulatory properties .
Synthesis Analysis
The Synthesis and Evaluation of Pironetin and Pironetin Analogs as Ovarian Cancer Chemotherapeutic Agents was discussed in a dissertation . The synthesis involved Alkaloid-catalyzed acyl halide-aldehyde cyclocondensation (AAC) reactions in an asymmetric total synthesis of both the natural (−) and unnatural (+) antipodes of pironetin .Molecular Structure Analysis
Pironetin forms a covalent bond to cysteine-316 in α-tubulin via a Michael addition reaction . The crystal structure of the tubulin-pironetin complex revealed that pironetin perturbs the T7 loop and helix H8 of α-tubulin .Chemical Reactions Analysis
Pironetin reacts covalently with cysteine-316 of α-tubulin to destabilize microtubule . The Alkaloid-catalyzed acyl halide-aldehyde cyclocondensation (AAC) reactions were used in an asymmetric total synthesis of both the natural (−) and unnatural (+) antipodes of pironetin .Physical And Chemical Properties Analysis
Pironetin has a molecular formula of C19H32O4, an average mass of 324.455 Da, and a mono-isotopic mass of 324.230072 Da . It has a density of 1.0±0.1 g/cm3, a boiling point of 473.1±40.0 °C at 760 mmHg, and a vapour pressure of 0.0±2.7 mmHg at 25°C .Scientific Research Applications
Cancer Treatment: Microtubule Polymerization Inhibitor
Pironetin is well-known for its role as a microtubule polymerization inhibitor . It binds covalently to α-tubulin at cysteine-316, which destabilizes microtubule dynamics . This action halts the cell cycle in the M phase, making pironetin a potent anticancer agent with an IC50 in the range of 1.5–26 nM . Its ability to disrupt microtubules is crucial for cancer therapeutics, as it can inhibit tumor growth significantly.
Antiproliferative Activity
The antiproliferative activity of pironetin against cultured cancer cells is another key application. By binding to α-tubulin, pironetin prevents the normal dynamic processes of tubulin polymerization, which are essential for cell division and growth . This makes it a valuable tool for research into cell cycle regulation and cancer cell proliferation.
Structural Biology and Drug Design
Pironetin’s interaction with α-tubulin provides a basis for the rational design of chemotherapeutics targeting α-tubulin . Structural biology can utilize the crystallographic data of pironetin bound to α-tubulin to design new analogs with improved efficacy and reduced resistance in cancer treatment.
Molecular Docking Studies
Molecular docking studies of pironetin analogues, such as cryptoconcatones, have been conducted to understand their binding capacity to α-tubulin . These studies help in delineating structure–binding relationships and contribute to the design of new compounds with targeted action against cancer cells.
Understanding Mechanisms of Action
Research into the mechanisms of action of compounds structurally similar to pironetin, like cryptoconcatones, is facilitated by pironetin’s known binding properties . This aids in a better understanding of how these compounds exert their antiproliferative effects, which is vital for developing new anticancer strategies.
Comparative Analysis with Other MTAs
Pironetin serves as a comparative agent against other microtubule-targeting agents (MTAs) like vinblastine and taxol . By understanding the unique binding site and mechanism of pironetin, researchers can explore alternative pathways for drug development that might circumvent the resistance often developed against β-tubulin-targeting drugs.
Mechanism of Action
Target of Action
Pironetin is a natural product that primarily targets α-tubulin , a protein that is a key component of microtubules . Microtubules are dynamic structures that play crucial roles in various cellular processes, including cell division . Pironetin specifically binds to the Cysteine-316 residue of α-tubulin .
Mode of Action
Pironetin interacts with its target, α-tubulin, by forming a covalent bond with the Cysteine-316 residue . This covalent bonding is achieved through a Michael addition reaction . The binding of Pironetin to α-tubulin perturbs the T7 loop and helix H8 of α-tubulin . These elements are essential for establishing longitudinal tubulin contacts in microtubules .
Future Directions
properties
IUPAC Name |
(2R,3R)-3-ethyl-2-[(E,2R,3S,4R,5S)-2-hydroxy-4-methoxy-3,5-dimethylnon-7-enyl]-2,3-dihydropyran-6-one | |
---|---|---|
Source | PubChem | |
URL | https://pubchem.ncbi.nlm.nih.gov | |
Description | Data deposited in or computed by PubChem | |
InChI |
InChI=1S/C19H32O4/c1-6-8-9-13(3)19(22-5)14(4)16(20)12-17-15(7-2)10-11-18(21)23-17/h6,8,10-11,13-17,19-20H,7,9,12H2,1-5H3/b8-6+/t13-,14-,15+,16+,17+,19+/m0/s1 | |
Source | PubChem | |
URL | https://pubchem.ncbi.nlm.nih.gov | |
Description | Data deposited in or computed by PubChem | |
InChI Key |
XIHGDBYGUWEHCV-FSEPSNHWSA-N | |
Source | PubChem | |
URL | https://pubchem.ncbi.nlm.nih.gov | |
Description | Data deposited in or computed by PubChem | |
Canonical SMILES |
CCC1C=CC(=O)OC1CC(C(C)C(C(C)CC=CC)OC)O | |
Source | PubChem | |
URL | https://pubchem.ncbi.nlm.nih.gov | |
Description | Data deposited in or computed by PubChem | |
Isomeric SMILES |
CC[C@@H]1C=CC(=O)O[C@@H]1C[C@H]([C@H](C)[C@@H]([C@@H](C)C/C=C/C)OC)O | |
Source | PubChem | |
URL | https://pubchem.ncbi.nlm.nih.gov | |
Description | Data deposited in or computed by PubChem | |
Molecular Formula |
C19H32O4 | |
Source | PubChem | |
URL | https://pubchem.ncbi.nlm.nih.gov | |
Description | Data deposited in or computed by PubChem | |
DSSTOX Substance ID |
DTXSID601037185 | |
Record name | Pironetin | |
Source | EPA DSSTox | |
URL | https://comptox.epa.gov/dashboard/DTXSID601037185 | |
Description | DSSTox provides a high quality public chemistry resource for supporting improved predictive toxicology. | |
Molecular Weight |
324.5 g/mol | |
Source | PubChem | |
URL | https://pubchem.ncbi.nlm.nih.gov | |
Description | Data deposited in or computed by PubChem | |
Product Name |
Pironetin | |
CAS RN |
151519-02-7 | |
Record name | Pironetin | |
Source | ChemIDplus | |
URL | https://pubchem.ncbi.nlm.nih.gov/substance/?source=chemidplus&sourceid=0151519027 | |
Description | ChemIDplus is a free, web search system that provides access to the structure and nomenclature authority files used for the identification of chemical substances cited in National Library of Medicine (NLM) databases, including the TOXNET system. | |
Record name | Pironetin | |
Source | EPA DSSTox | |
URL | https://comptox.epa.gov/dashboard/DTXSID601037185 | |
Description | DSSTox provides a high quality public chemistry resource for supporting improved predictive toxicology. | |
Retrosynthesis Analysis
AI-Powered Synthesis Planning: Our tool employs the Template_relevance Pistachio, Template_relevance Bkms_metabolic, Template_relevance Pistachio_ringbreaker, Template_relevance Reaxys, Template_relevance Reaxys_biocatalysis model, leveraging a vast database of chemical reactions to predict feasible synthetic routes.
One-Step Synthesis Focus: Specifically designed for one-step synthesis, it provides concise and direct routes for your target compounds, streamlining the synthesis process.
Accurate Predictions: Utilizing the extensive PISTACHIO, BKMS_METABOLIC, PISTACHIO_RINGBREAKER, REAXYS, REAXYS_BIOCATALYSIS database, our tool offers high-accuracy predictions, reflecting the latest in chemical research and data.
Strategy Settings
Precursor scoring | Relevance Heuristic |
---|---|
Min. plausibility | 0.01 |
Model | Template_relevance |
Template Set | Pistachio/Bkms_metabolic/Pistachio_ringbreaker/Reaxys/Reaxys_biocatalysis |
Top-N result to add to graph | 6 |
Feasible Synthetic Routes
Disclaimer and Information on In-Vitro Research Products
Please be aware that all articles and product information presented on BenchChem are intended solely for informational purposes. The products available for purchase on BenchChem are specifically designed for in-vitro studies, which are conducted outside of living organisms. In-vitro studies, derived from the Latin term "in glass," involve experiments performed in controlled laboratory settings using cells or tissues. It is important to note that these products are not categorized as medicines or drugs, and they have not received approval from the FDA for the prevention, treatment, or cure of any medical condition, ailment, or disease. We must emphasize that any form of bodily introduction of these products into humans or animals is strictly prohibited by law. It is essential to adhere to these guidelines to ensure compliance with legal and ethical standards in research and experimentation.