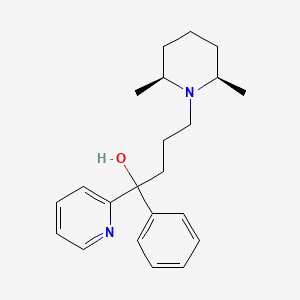
Pirmenol
Overview
Description
Pirmenol is a Class Ia antiarrhythmic agent with quinidine-like properties, primarily targeting voltage-gated sodium channels to suppress abnormal cardiac rhythms. It also exhibits inhibitory effects on potassium and calcium channels, contributing to its ability to prolong the effective refractory period and stabilize myocardial cell membranes . Clinically, it is used to manage ventricular arrhythmias, including drug-resistant ventricular tachycardia (VT) and premature ventricular contractions (PVCs). This compound demonstrates high oral bioavailability (~87%), a half-life of 6–9 hours, and dose-dependent efficacy with minimal hemodynamic side effects . Its stereoisomeric properties and metabolite activity further differentiate it from other antiarrhythmics .
Preparation Methods
Synthetic Routes and Reaction Conditions: Pirmenol hydrochloride is a pyridinemethanol derivative. The synthesis involves the reaction of pyridine with various reagents to form the desired compound. The specific synthetic route includes the following steps:
Formation of the Pyridinemethanol Core: The core structure is synthesized by reacting pyridine with formaldehyde and a suitable reducing agent.
Substitution Reactions: The core structure undergoes substitution reactions with different alkylating agents to introduce the necessary functional groups.
Purification: The final product is purified using recrystallization or chromatography techniques to obtain pure this compound hydrochloride.
Industrial Production Methods: Industrial production of this compound involves large-scale synthesis using the same principles as the laboratory synthesis but optimized for higher yields and purity. The process includes:
Bulk Synthesis: Large reactors are used to carry out the reactions under controlled conditions.
Purification: Industrial-scale purification techniques such as distillation, crystallization, and chromatography are employed.
Quality Control: Rigorous quality control measures are in place to ensure the final product meets the required standards.
Chemical Reactions Analysis
Types of Reactions: Pirmenol undergoes various chemical reactions, including:
Oxidation: this compound can be oxidized to form quinone derivatives.
Reduction: Reduction reactions can convert quinone derivatives back to hydroquinones.
Substitution: Electrophilic aromatic substitution reactions can occur on the aromatic ring of this compound.
Common Reagents and Conditions:
Oxidation: Common oxidizing agents include potassium permanganate and chromium trioxide.
Reduction: Reducing agents such as sodium borohydride and lithium aluminum hydride are used.
Substitution: Electrophilic reagents like halogens and nitrating agents are used under acidic conditions.
Major Products:
Oxidation Products: Quinone derivatives.
Reduction Products: Hydroquinones.
Substitution Products: Halogenated or nitrated derivatives of this compound.
Scientific Research Applications
Pirmenol has several scientific research applications, including:
Chemistry: Used as a model compound to study the effects of antiarrhythmic agents on cardiac cells.
Biology: Investigated for its effects on ion channels and cellular electrophysiology.
Medicine: Used in clinical trials to evaluate its efficacy and safety in treating arrhythmias.
Industry: Employed in the development of new antiarrhythmic drugs and formulations
Mechanism of Action
Pirmenol exerts its effects by blocking sodium and potassium channels in cardiac cells. This action prolongs the action potential duration and reduces the excitability of the cardiac cells, thereby preventing arrhythmias . The molecular targets include the fast sodium channels and the delayed rectifying potassium channels. The drug binds to these channels and inhibits their function, leading to a decrease in the rate of depolarization and repolarization .
Comparison with Similar Compounds
Comparison with Class Ia Antiarrhythmic Agents
Procainamide
- Efficacy: In drug-resistant VT patients, pirmenol (1.1 mg/kg IV) reduced inducible VT rates from 221 to 166 bpm, outperforming procainamide (1000 mg IV), which only slowed VT to 200 bpm. This compound also showed superior VT suppression (10/12 patients) compared to procainamide (1/9 patients) .
- Pharmacokinetics (PK): this compound’s longer half-life (7–9.4 hours vs. procainamide’s 2.5–4.7 hours) allows less frequent dosing .
Quinidine
- Mechanistic Advantage: this compound’s antiarrhythmic effects are less dependent on serum potassium levels, reducing the risk of hypokalemia-induced inefficacy—a limitation observed with quinidine .
- Efficacy in Models: Both drugs show high efficacy in coronary-ligated rats, but this compound achieves protection at lower plasma concentrations (1.2 µg/mL vs. quinidine’s 5 mg/kg dose) .
Disopyramide
- Proarrhythmic Risk: this compound has a lower propensity to exacerbate reentrant arrhythmias in post-myocardial infarction models compared to disopyramide .
- PK Profile: this compound’s oral bioavailability (87%) exceeds disopyramide’s (70–80%), and its protein binding (83–90%) is comparable .
Comparison with Other Antiarrhythmic Classes
Class Ib (e.g., Lidocaine, Mexiletine)
- Sodium Channel Blockade: this compound (Class Ia) exhibits stronger use-dependent sodium channel blockade than mexiletine (Class Ib), making it more effective in suppressing high-frequency ectopic activity .
- Clinical Use: Unlike lidocaine (IV-only), this compound is effective orally, with 70–150 mg doses achieving >90% PVC suppression .
Class Ic (e.g., Pilsicainide)
- Potency: this compound (1 mM) blocks sodium currents (INa) as effectively as pilsicainide but with a wider therapeutic index in ischemia models .
- Proarrhythmia: this compound’s clinical proarrhythmia rate (<2%) is lower than that of Class Ic agents like flecainide, which carry a higher risk of lethal VT .
Proarrhythmic Potential
This compound’s proarrhythmic effects are context-dependent. In dogs with small myocardial infarcts, it facilitated VT induction by increasing repolarization dispersion . However, clinical trials reported low proarrhythmia rates (2–6%), comparable to lidocaine but safer than quinidine in acute MI settings .
Pharmacokinetic Advantages
Parameter | This compound | Procainamide | Quinidine |
---|---|---|---|
Bioavailability | 87% | 75–95% | 70–80% |
Half-life (h) | 6–9 | 2.5–4.7 | 6–8 |
Protein Binding | 83–90% | 15–20% | 80–90% |
Dosing Frequency | Twice daily | 4–6 times daily | 3–4 times daily |
This compound’s prolonged half-life and consistent absorption reduce dosing frequency, improving patient compliance .
Stereoisomeric and Metabolite Considerations
- Enantiomers: The (-)-enantiomer has 49% higher unbound clearance than (+)-pirmenol, but both show equivalent antiarrhythmic potency in PVC suppression (EC90: ~1.5 µg/mL) .
- This dual activity may enhance efficacy in heterogeneous arrhythmias .
Adverse Effect Profile
Side Effect | This compound | Procainamide | Quinidine |
---|---|---|---|
Hypotension | Rare | Common | Common |
GI Disturbances | Mild | Moderate | Severe |
Proarrhythmia | Low | Moderate | High |
Taste Disturbance | Common | None | None |
This compound’s most frequent side effects—bitter taste and dry mouth—are benign compared to quinidine’s anticholinergic effects or procainamide’s lupus risk .
Biological Activity
Pirmenol is an antiarrhythmic agent classified primarily as a Class IA drug, known for its unique electrophysiological properties and therapeutic applications in managing various cardiac arrhythmias. This article explores the biological activity of this compound, focusing on its mechanisms of action, pharmacokinetics, clinical efficacy, and comparative studies with other antiarrhythmic medications.
This compound functions by blocking specific ion channels and receptors involved in cardiac action potentials. Its primary mechanism includes:
- Inhibition of IK.ACh Channels : this compound inhibits the muscarinic acetylcholine receptor (mAchR) which leads to a reduction in the inward potassium current (IK.ACh) with an IC50 value of 0.1 μM. This inhibition is crucial for its antiarrhythmic effects, particularly in atrial cells .
- Action Potential Modulation : At concentrations of 1 μM, this compound prolongs the action potential duration (APD) in atrial muscles and Purkinje fibers, affecting the early plateau and final repolarization phases in ventricular myocytes .
Pharmacokinetics
The pharmacokinetic profile of this compound reveals significant differences between its enantiomers:
- Enantiomeric Differences : The study of this compound's enantiomers shows that (-)-pirmenol has approximately 47% lower clearance and a 33% lower steady-state distribution volume compared to (+)-pirmenol. These differences are attributed to stereoselective plasma protein binding, which affects their pharmacodynamic properties .
- Plasma Concentration Dynamics : Following administration, plasma concentrations of total (-)-pirmenol were found to exceed those of (+)-pirmenol. The area under the curve (AUC) values indicated that unbound concentrations were more comparable between the two enantiomers, suggesting that both are effective in arrhythmia suppression despite their pharmacokinetic variances .
Clinical Efficacy
Clinical studies have demonstrated the effectiveness of this compound in treating arrhythmias:
- Antiarrhythmic Effectiveness : In a study involving 16 patients with frequent ventricular ectopic depolarizations, this compound was shown to be effective in suppressing arrhythmias with minimal adverse effects. The therapeutic plasma concentrations were well-tolerated by patients .
- Comparative Studies : When compared with disopyramide, this compound exhibited more potent negative dromotropic effects on PQ interval and QRS width while inducing modest hypotension. This suggests that this compound may offer advantages over traditional antiarrhythmic medications in certain clinical scenarios .
Case Studies
Several case studies highlight the clinical application of this compound:
- Case Study on Ventricular Ectopic Depolarizations : A randomized study found that this compound effectively suppressed arrhythmias in patients who did not respond well to quinidine. The mean change in QT interval was significantly less with this compound compared to quinidine, indicating a favorable side effect profile .
- Pharmacodynamics in Dogs : Research conducted on canine models demonstrated that intravenous administration of this compound led to transient increases in coronary blood flow followed by a decrease, showcasing its complex cardiovascular effects .
Summary Table of Key Findings
Feature | This compound | Disopyramide |
---|---|---|
Class | IA | IA |
Primary Mechanism | IK.ACh inhibition | Fast inward sodium current inhibition |
Negative Dromotropic Effect | More potent | Less potent |
Hypotensive Effect | Modest | Increased blood pressure |
QT Interval Change | Minimal | Significant increase |
Q & A
Basic Research Questions
Q. What experimental models are most suitable for studying Pirmenol's antiarrhythmic mechanisms?
- Methodology : Use isolated guinea pig atrial myocytes to evaluate this compound's inhibition of carbachol-induced IK.ACh currents. Measure concentration-dependent effects (e.g., IC50 = 0.1 μM for carbachol-induced IK.ACh inhibition) via voltage-clamp techniques . Validate findings in Langendorff-perfused heart preparations to assess atrial fibrillation threshold modulation . Rodent models (mice/rats) are ideal for acute oral/IV pharmacokinetic studies due to established dosing protocols (e.g., 2.5% aqueous solution for oral administration in rats) .
Q. How does this compound’s selectivity for muscarinic receptors compare to other antiarrhythmic agents?
- Methodology : Conduct competitive binding assays using radiolabeled ligands (e.g., [³H]-QNB) to quantify receptor affinity. Compare this compound’s inhibition of GTPγS-induced currents (IC50 = 30 μM) with agents like atropine. Note that this compound’s reversible inhibition of IK.ACh suggests partial selectivity for acetylcholine receptor-operated K+ channels over direct GTPγS pathways .
Q. What are the critical parameters for ensuring this compound’s stability in in vitro assays?
- Methodology : Store this compound hydrochloride at -20°C in powder form (stable for ≥2 years) or dissolved in sterile aqueous solutions under nitrogen (-80°C for 6 months; -20°C for 1 month). Validate purity via HPLC (≥97.2%) and monitor degradation using UV-Vis spectroscopy .
Advanced Research Questions
Q. How can researchers resolve contradictions in this compound’s efficacy across species (e.g., guinea pigs vs. dogs)?
- Methodology : Perform cross-species pharmacokinetic profiling. For example, in dogs, administer this compound via infusion pump (1 mg/kg/min) and compare plasma concentration curves with guinea pig atrial cell data. Adjust for species-specific differences in cytochrome P450 metabolism using liver microsome assays .
Q. What statistical approaches are optimal for analyzing this compound’s dose-response variability in heterogeneous populations?
- Methodology : Apply mixed-effects models to account for inter-subject variability in rodent/dog studies. Use Bayesian hierarchical models to integrate prior data (e.g., IC50 ranges) and reduce uncertainty in small-sample studies .
Q. How can this compound’s formulation be optimized for long-term preclinical trials?
- Methodology : Test lyophilized formulations for enhanced shelf-life. Use differential scanning calorimetry (DSC) to assess excipient compatibility. For oral dosing in dogs, encapsulate this compound in gelatin capsules to prevent hydrolysis; validate bioavailability via LC-MS/MS .
Q. What safety protocols are essential for handling this compound in genotoxicity studies?
- Methodology : Follow SDS guidelines for PPE (gloves, lab coats) and conduct Ames tests to assess mutagenicity. Reference prior genotoxicity data showing this compound’s non-mutagenic profile at therapeutic doses (e.g., 30 μM in bacterial reverse mutation assays) .
Q. Data Reproducibility & Validation
Q. How can researchers ensure reproducibility of this compound’s IK.ACh inhibition assays?
- Methodology : Document experimental conditions rigorously:
- Solution composition : 0.9% NaCl, pH 7.4, 37°C.
- Electrophysiology settings : Holding potential -50 mV, 300 ms depolarizing pulses .
- Share raw data and protocols via repositories (e.g., Zenodo) with DOIs .
Q. What steps mitigate batch-to-batch variability in this compound hydrochloride?
- Methodology : Source batches from certified suppliers (e.g., Ambeed Inc.) with ≥97% purity. Perform NMR/Fourier-transform infrared (FTIR) spectroscopy to confirm structural consistency. Include batch numbers in metadata .
Q. Integration with Emerging Technologies
Q. Can machine learning predict this compound’s interactions with novel cardiac ion channels?
Properties
IUPAC Name |
4-[(2S,6R)-2,6-dimethylpiperidin-1-yl]-1-phenyl-1-pyridin-2-ylbutan-1-ol | |
---|---|---|
Source | PubChem | |
URL | https://pubchem.ncbi.nlm.nih.gov | |
Description | Data deposited in or computed by PubChem | |
InChI |
InChI=1S/C22H30N2O/c1-18-10-8-11-19(2)24(18)17-9-15-22(25,20-12-4-3-5-13-20)21-14-6-7-16-23-21/h3-7,12-14,16,18-19,25H,8-11,15,17H2,1-2H3/t18-,19+,22? | |
Source | PubChem | |
URL | https://pubchem.ncbi.nlm.nih.gov | |
Description | Data deposited in or computed by PubChem | |
InChI Key |
APUDBKTWDCXQJA-QIDMFYOTSA-N | |
Source | PubChem | |
URL | https://pubchem.ncbi.nlm.nih.gov | |
Description | Data deposited in or computed by PubChem | |
Canonical SMILES |
CC1CCCC(N1CCCC(C2=CC=CC=C2)(C3=CC=CC=N3)O)C | |
Source | PubChem | |
URL | https://pubchem.ncbi.nlm.nih.gov | |
Description | Data deposited in or computed by PubChem | |
Isomeric SMILES |
C[C@@H]1CCC[C@@H](N1CCCC(C2=CC=CC=C2)(C3=CC=CC=N3)O)C | |
Source | PubChem | |
URL | https://pubchem.ncbi.nlm.nih.gov | |
Description | Data deposited in or computed by PubChem | |
Molecular Formula |
C22H30N2O | |
Source | PubChem | |
URL | https://pubchem.ncbi.nlm.nih.gov | |
Description | Data deposited in or computed by PubChem | |
DSSTOX Substance ID |
DTXSID1043839 | |
Record name | Pirmenol | |
Source | EPA DSSTox | |
URL | https://comptox.epa.gov/dashboard/DTXSID1043839 | |
Description | DSSTox provides a high quality public chemistry resource for supporting improved predictive toxicology. | |
Molecular Weight |
338.5 g/mol | |
Source | PubChem | |
URL | https://pubchem.ncbi.nlm.nih.gov | |
Description | Data deposited in or computed by PubChem | |
CAS No. |
68252-19-7, 129885-18-3, 129885-19-4 | |
Record name | Pirmenol | |
Source | CAS Common Chemistry | |
URL | https://commonchemistry.cas.org/detail?cas_rn=68252-19-7 | |
Description | CAS Common Chemistry is an open community resource for accessing chemical information. Nearly 500,000 chemical substances from CAS REGISTRY cover areas of community interest, including common and frequently regulated chemicals, and those relevant to high school and undergraduate chemistry classes. This chemical information, curated by our expert scientists, is provided in alignment with our mission as a division of the American Chemical Society. | |
Explanation | The data from CAS Common Chemistry is provided under a CC-BY-NC 4.0 license, unless otherwise stated. | |
Record name | (+)-Pirmenol | |
Source | ChemIDplus | |
URL | https://pubchem.ncbi.nlm.nih.gov/substance/?source=chemidplus&sourceid=0129885183 | |
Description | ChemIDplus is a free, web search system that provides access to the structure and nomenclature authority files used for the identification of chemical substances cited in National Library of Medicine (NLM) databases, including the TOXNET system. | |
Record name | (-)-Pirmenol | |
Source | ChemIDplus | |
URL | https://pubchem.ncbi.nlm.nih.gov/substance/?source=chemidplus&sourceid=0129885194 | |
Description | ChemIDplus is a free, web search system that provides access to the structure and nomenclature authority files used for the identification of chemical substances cited in National Library of Medicine (NLM) databases, including the TOXNET system. | |
Record name | Pirmenol | |
Source | EPA DSSTox | |
URL | https://comptox.epa.gov/dashboard/DTXSID1043839 | |
Description | DSSTox provides a high quality public chemistry resource for supporting improved predictive toxicology. | |
Retrosynthesis Analysis
AI-Powered Synthesis Planning: Our tool employs the Template_relevance Pistachio, Template_relevance Bkms_metabolic, Template_relevance Pistachio_ringbreaker, Template_relevance Reaxys, Template_relevance Reaxys_biocatalysis model, leveraging a vast database of chemical reactions to predict feasible synthetic routes.
One-Step Synthesis Focus: Specifically designed for one-step synthesis, it provides concise and direct routes for your target compounds, streamlining the synthesis process.
Accurate Predictions: Utilizing the extensive PISTACHIO, BKMS_METABOLIC, PISTACHIO_RINGBREAKER, REAXYS, REAXYS_BIOCATALYSIS database, our tool offers high-accuracy predictions, reflecting the latest in chemical research and data.
Strategy Settings
Precursor scoring | Relevance Heuristic |
---|---|
Min. plausibility | 0.01 |
Model | Template_relevance |
Template Set | Pistachio/Bkms_metabolic/Pistachio_ringbreaker/Reaxys/Reaxys_biocatalysis |
Top-N result to add to graph | 6 |
Feasible Synthetic Routes
Disclaimer and Information on In-Vitro Research Products
Please be aware that all articles and product information presented on BenchChem are intended solely for informational purposes. The products available for purchase on BenchChem are specifically designed for in-vitro studies, which are conducted outside of living organisms. In-vitro studies, derived from the Latin term "in glass," involve experiments performed in controlled laboratory settings using cells or tissues. It is important to note that these products are not categorized as medicines or drugs, and they have not received approval from the FDA for the prevention, treatment, or cure of any medical condition, ailment, or disease. We must emphasize that any form of bodily introduction of these products into humans or animals is strictly prohibited by law. It is essential to adhere to these guidelines to ensure compliance with legal and ethical standards in research and experimentation.