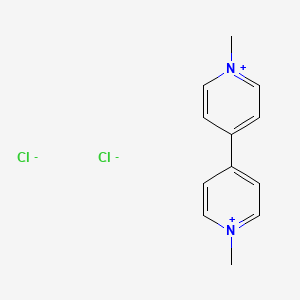
Paraquat dichloride
Overview
Description
Paraquat dichloride (C₁₂H₁₄Cl₂N₂) is a bipyridyl herbicide first synthesized in 1882 as methyl viologen, a chemical dye . Its herbicidal properties were discovered in 1955, leading to commercialization in 1962 under the brand name Gramoxone . It is a non-selective, contact herbicide that rapidly desiccates green plant tissues by disrupting photosynthesis via reactive oxygen species (ROS) generation .
Preparation Methods
Synthetic Routes and Reaction Conditions: Paraquat dichloride is synthesized through the coupling of pyridine, which is treated with sodium in ammonia, followed by oxidation to yield 4,4′-bipyridine. This intermediate is then dimethylated using chloromethane to produce the final product as the dichloride salt .
Industrial Production Methods: In industrial settings, this compound is produced in large quantities using similar synthetic routes. The process involves the careful control of reaction conditions to ensure high yield and purity. The final product is often formulated into various concentrations and forms, such as aqueous solutions and water-soluble granules, for agricultural use .
Chemical Reactions Analysis
Types of Reactions: Paraquat dichloride undergoes several types of chemical reactions, including:
Substitution: this compound can react with strong acids, bases, and oxidizing agents, leading to various substitution reactions.
Common Reagents and Conditions:
Oxidation: Common reagents include molecular oxygen and electron donors like NADPH.
Reduction: Sodium dithionite is often used to reduce this compound in analytical methods.
Substitution: Strong acids and bases are used under controlled conditions to facilitate substitution reactions.
Major Products: The major products formed from these reactions include superoxide radicals and other reactive oxygen species, which are responsible for the compound’s herbicidal activity .
Scientific Research Applications
Agricultural Applications
Paraquat dichloride is primarily employed as a broad-spectrum herbicide to control a wide range of grasses and broad-leaved weeds. Its fast-acting nature makes it particularly useful in various agricultural settings, including:
- Crop Management : It is utilized in the cultivation of crops such as oil palm, where it helps manage weed growth effectively, thereby enhancing crop yield. A study conducted in an oil palm plantation demonstrated that different application rates of this compound significantly influenced soil pH and permeability but did not adversely affect soil bulk density or porosity .
- Pre-harvest Desiccation : Paraquat is often used to desiccate crops before harvest, allowing for easier harvesting and improved crop quality.
Table 1: Effects of this compound on Soil Properties
Treatment | pH Level | Soil Permeability | Total Nitrogen Content |
---|---|---|---|
Control | 5.8 | 0.12 cm/s | 0.15% |
4 ml/l | 6.2 | 0.15 cm/s | 0.10% |
8 ml/l | 5.6 | 0.10 cm/s | 0.08% |
16 ml/l | 5.5 | 0.11 cm/s | 0.09% |
Environmental Impact Studies
Research has shown that this compound can have significant ecological effects, particularly on aquatic ecosystems due to runoff from agricultural fields. Studies have documented its impact on aquatic organisms, including fish species like Channa punctatus, where exposure led to notable histopathological changes in vital organs such as gills, liver, and kidneys . This underscores the importance of monitoring paraquat levels in water bodies to protect aquatic life.
Case Study: Histopathological Effects on Fish
A study evaluated the effects of this compound on freshwater fish exposed to varying concentrations over a period of time:
- Acute Exposure : Fish exposed to a concentration of for 96 hours exhibited significant gill damage, including curling of secondary lamellae and congestion .
- Chronic Effects : Prolonged exposure resulted in liver necrosis and kidney damage, indicating the compound's potential to cause long-term harm to aquatic organisms.
Toxicological Research
This compound is also a subject of toxicological studies due to its high toxicity levels in humans and animals upon ingestion or dermal exposure. It is associated with severe health risks, including pulmonary fibrosis and renal failure.
Case Study: Paraquat Poisoning
A case series reported on patients who ingested this compound with suicidal intent:
- Patient Outcomes : One patient ingested and required intensive care management involving continuous venovenous hemofiltration due to acute kidney injury . Another patient showed significant recovery after aggressive treatment despite severe initial symptoms .
Health Risks and Epidemiological Studies
Epidemiological studies have linked paraquat exposure to increased risks of neurodegenerative diseases such as Parkinson's disease (PD). Research conducted in agricultural regions indicated that individuals with higher ambient exposure levels had significantly elevated odds of developing PD .
Table 2: Risk Factors for Parkinson's Disease Associated with Paraquat Exposure
Exposure Level | Odds Ratio (OR) | Confidence Interval (CI) |
---|---|---|
Residential Proximity | 1.91 | (1.30, 2.83) |
Workplace Proximity | 2.15 | (1.46, 3.19) |
Mechanism of Action
Paraquat dichloride exerts its herbicidal effects by interfering with the photosynthetic process in plants. It accepts electrons from photosystem I and transfers them to molecular oxygen, producing reactive oxygen species. These reactive oxygen species cause oxidative stress, leading to the destruction of cell membranes and rapid plant tissue death . In humans and animals, this compound’s toxicity is primarily due to its ability to generate reactive oxygen species, causing damage to vital organs such as the lungs, liver, and kidneys .
Comparison with Similar Compounds
Chemical and Physical Properties
- Water solubility : 620 g/L at 20°C, enabling rapid absorption by foliage and soil .
- Soil adsorption : Strong ionic binding to clay and organic particles, reducing leaching but increasing persistence in soil .
- Volatility : Negligible vapor pressure (<0.1 mPa), minimizing airborne dispersion .
Uses
Paraquat dichloride is applied in agriculture for weed control in crops (e.g., oil palm, rubber, rice) and non-cropland . Its rapid action and soil immobilization make it suitable for pre-harvest desiccation and pasture restoration .
Toxicity
- Human toxicity : Acute exposure causes multi-organ failure, while chronic exposure is linked to pulmonary fibrosis and Parkinson’s disease via oxidative stress and α-synuclein aggregation .
- Ecotoxicity : Histopathological damage in fish (e.g., Channa punctatus) and arthropods, though field studies show minimal impact on soil ecosystems .
Regulatory Status
Classified as a WHO Group II "moderately hazardous" pesticide, paraquat is restricted in 58 countries due to its high acute toxicity .
Diquat: Structural and Functional Analog
Diquat (C₁₂H₁₂Br₂N₂) is another bipyridyl herbicide with a similar redox-driven mode of action but distinct toxicological and environmental profiles.
Table 1: this compound vs. Diquat
Key Differences :
- Paraquat’s lung specificity arises from active uptake by alveolar polyamine transporters, whereas diquat distributes systemically .
- Environmental persistence of paraquat is higher due to strong soil binding, whereas diquat may leach more readily .
MPP+: Structural Analog in Neurotoxicity
MPP+ (1-methyl-4-phenylpyridinium), a metabolite of the neurotoxin MPTP, shares structural homology with paraquat. Both compounds induce mitochondrial dysfunction and oxidative stress, but MPP+ specifically inhibits complex I in dopaminergic neurons, directly modeling Parkinson’s pathology .
Table 2: this compound vs. MPP+
Research Findings and Contradictions
- Parkinson’s Disease : Epidemiological studies report strong correlations between paraquat exposure and Parkinson’s, but mechanistic differences from MPP+ (e.g., lack of complex I inhibition) suggest alternative pathways .
Biological Activity
Paraquat dichloride is a widely used herbicide known for its effectiveness in controlling weeds. However, its biological activity raises significant concerns due to its toxicity and potential health risks, particularly in agricultural settings. This article explores the biological effects of this compound, focusing on its mechanisms of action, associated health risks, and case studies highlighting its impact on human health.
Paraquat exerts its toxic effects primarily through the generation of reactive oxygen species (ROS), leading to oxidative stress. This oxidative stress can cause significant cellular damage, including:
- DNA Damage : Studies have shown that paraquat induces dose-dependent DNA damage in peripheral lymphocyte cells, as evidenced by alkaline comet assays and other cytotoxicity tests .
- Cell Membrane Integrity : Exposure to paraquat compromises cell membrane integrity, resulting in increased cell permeability and eventual cell death .
- Organ Toxicity : Paraquat has been linked to pathological changes in vital organs such as the lungs, kidneys, and liver. Histopathological examinations reveal significant tissue damage following paraquat exposure .
Table 1: Summary of Biological Effects of this compound
Biological Effect | Mechanism | Evidence Source |
---|---|---|
Oxidative Stress | ROS generation | |
DNA Damage | Alkaline comet assay | |
Cell Membrane Damage | Increased permeability | |
Organ Toxicity | Histopathological changes |
Health Risks
Paraquat exposure is associated with several health risks, particularly in agricultural workers. Epidemiological studies indicate a strong correlation between paraquat exposure and the development of Parkinson's disease (PD). A recent population-based study in California found that individuals living or working near areas with high paraquat usage had significantly increased odds of developing PD .
Case Studies
- Case Study on Acute Poisoning :
- Long-term Effects :
Research Findings
Recent research emphasizes the need for further investigation into the long-term effects of paraquat exposure. A systematic review concluded that paraquat is a significant risk factor for Parkinson's disease, particularly among individuals exposed at younger ages . The study highlighted that ambient exposure levels correlate with increased disease incidence, reinforcing the need for regulatory scrutiny.
Table 2: Epidemiological Findings on Paraquat and Parkinson's Disease
Q & A
Basic Research Questions
Q. What standardized experimental protocols are recommended for assessing the hydrolysis stability of Paraquat dichloride in environmental samples?
Hydrolysis studies should be conducted at pH 5, 7, and 9 under controlled temperatures (e.g., 25°C and 40°C) to evaluate stability. Data from the EPA indicate no hydrolysis occurs under these conditions, even after prolonged incubation . Buffered aqueous solutions should be used, and degradation products analyzed via TLC or HPLC.
Q. How should researchers design studies to evaluate this compound adsorption in soil and water systems?
Batch experiments with controlled variables (e.g., adsorbent dose, pH, initial concentration) are critical. For example, activated date stone carbon (8.0 g/L) achieved equilibrium adsorption in 140 minutes at pH 9.0. Kinetic analysis should use pseudo-second-order models, validated by regression analysis .
Q. What regulatory data requirements must be addressed for this compound reregistration?
The EPA mandates product chemistry data (e.g., hydrolysis, photodegradation) and ecotoxicological studies (e.g., aerobic soil metabolism). Required studies are detailed in the "Requirements Status and Registrant’s Response" (Attachment C of EPA documents), including degradation kinetics and soil binding properties .
Advanced Research Questions
Q. How can researchers resolve contradictions in epidemiological data linking this compound to Parkinson’s disease?
Meta-analyses should stratify studies by exposure duration, dosage, and confounding factors (e.g., occupational vs. environmental exposure). Longitudinal cohort studies with biomarkers (e.g., urinary Paraquat levels) and neuroimaging can strengthen causality. The EPA notes a "large body of epidemiology data" but emphasizes methodological heterogeneity .
Q. What advanced methods improve the photocatalytic degradation efficiency of this compound in contaminated water?
TiO₂-Fe nanocomposites synthesized via sol-gel methods enhance visible-light absorption, reducing crystal size and bandgap energy. Degradation under sunlight irradiation achieves higher efficiency compared to UV alone. XRD and DRS characterization validate catalyst performance .
Q. How should thermodynamic parameters be integrated into adsorption studies to predict this compound removal efficacy?
Calculate Gibbs free energy (ΔG°), enthalpy (ΔH°), and entropy (ΔS°) from isotherm models (Langmuir, Freundlich). Exothermic processes (ΔH° < 0) with negative ΔG° indicate spontaneity. For example, activated date stone carbon adsorption showed ΔG° = -4.2 kJ/mol at 30°C .
Q. What statistical approaches are optimal for analyzing dose-response relationships in this compound toxicity studies?
Use multivariable logistic regression to adjust for covariates (e.g., age, co-exposure to other pesticides). In thyroid cancer studies, single-pollutant models and cumulative exposure indices over 20-year periods identified Paraquat as a significant risk factor (p < 0.05) .
Q. Methodological Challenges and Solutions
Q. How can researchers address data gaps in this compound’s environmental persistence?
Conduct long-term photodegradation studies on soil surfaces (e.g., 85-week sunlight exposure) and quantify irreversible soil binding. EPA data show no degradation in sterile sandy loam soil after 180 days, with 93% radioactivity retained as parent compound .
Q. What experimental controls are essential for validating this compound’s interaction with co-applied herbicides?
Include dark controls and isotopic labeling to track chemical interactions. For example, dimethylamine salt of MCPA reduces Paraquat’s phytotoxicity via compound formation, validated by HPLC and biological activity assays .
Properties
Key on ui mechanism of action |
The innate immune response is important in paraquat-induced acute lung injury, but the exact pathways involved are not elucidated. The objectives of this study were to determine the specific role of the NLRP3 inflammasome in the process. Acute lung injury was induced by administering paraquat (PQ) intraperitoneally. NLRP3 inflammasome including NLRP3, ASC, and caspase-1 mRNA and protein expression in lung tissue and IL-1beta and IL-18 levels in BALF were detected at 4, 8, 24, and 72 hr after PQ administration in rats. Moreover, rats were pretreated with 10, 30, and 50 mg/kg NLRP3 inflammasome blocker glybenclamide, respectively, 1 h before PQ exposure. At 72 hr after PQ administration, lung histopathology changes, NLRP3, ASC, and caspase-1 protein expression, as well as secretion of cytokines including IL-1beta and IL-18 in BALF were investigated. The NLRP3 inflammasome including NLRP3, ASC, caspase-1 expression, and cytokines IL-1beta and IL-18 levels in PQ poisoning rats were significantly higher than that in the control group. NLRP3 inflammasome blocker glybenclamide pretreatment attenuated lung edema, inhibited the NLRP3, ASC, and caspase-1 activation, and reduced IL-1beta and IL-18 levels in BALF. In the in vitro experiments, IL-1beta and IL-18 secreted from RAW264.7 mouse macrophages treated with paraquat were attenuated by glybenclamide. In conclusion, paraquat can induce IL-1beta/IL-18 secretion via NLRP3-ASC-caspase-1 pathway, and the NLRP3 inflammasome is essential for paraquat-induced acute lung injury. BACKGROUND: Paraquat (PQ) poisoning is a lethal toxicological challenge that served as a disease model of acute lung injury and pulmonary fibrosis, but the mechanism is undetermined and no effective treatment has been discovered. METHODS AND FINDINGS: We demonstrated that PQ injures mitochondria and leads to mtDNA release. The mtDNA mediated PBMC recruitment and stimulated the alveolar epithelial cell production of TGF-beta1 in vitro. The levels of mtDNA in circulation and bronchial alveolar lavage fluid (BALF) were elevated in a mouse of PQ-induced lung injury. DNaseI could protect PQ-induced lung injury and significantly improved survival. Acute lung injury markers, such as TNFalpha, IL-1beta, and IL-6, and marker of fibrosis, collagen I, were downregulated in parallel with the elimination of mtDNA by DNaseI. These data indicate a possible mechanism for PQ-induced, mtDNA-mediated lung injury, which may be shared by other causes of lung injury, as suggested by the same protective effect of DNaseI in bleomycin-induced lung injury model. Interestingly, increased mtDNA in the BALF of patients with amyopathic dermatomyositis-interstitial lung disease can be appreciated. CONCLUSIONS: DNaseI targeting mtDNA may be a promising approach for the treatment of PQ-induced acute lung injury and pulmonary fibrosis that merits fast tracking through clinical trials. Paraquat (PQ), one of the most widely used herbicides, has been used for several decades in agriculture. Some studies suggest that PQ has effects on the immune system. Moreover, previous studies have shown that PQ imparted some immunosuppressive effects. In the present study, cytotoxicity assays using splenic NK cells from mice treated for 28 days with PQ (at 0.2, 1, and 5 mg/kg) were performed to determine whether PQ altered the function of NK cells. Given that PQ was expected to induce an immunosuppressive effect, it was hypothesized that a gene involved in cellular metal ion homeostasis, metallothionein-1 (MT-1), could play an important role in this outcome. This belief was based on the fact that MT1 encodes a protein responsible for zinc ion homeostasis, and that a reduction in free zinc ion levels impairs NK cell function. The results showed that PQ treatments led to increased MT expression in several organs (liver, kidneys, testes) and in splenocytes, caused a reduction of both free zinc ions in sera and in free intracellular zinc, and reduced the expression of GATA-3, a zinc-finger transcription factor important for maturation and activity of T-cells and NK cells. These results provide a basis for a new molecular mechanism to describe potential immunosuppressive effects of PQ in vivo. Paraquat (PQ) causes selective degeneration of dopaminergic neurons in the substantia nigra pars compacta, reproducing an important pathological feature of Parkinson disease. Oxidative stress, c-Jun N-terminal kinase activation, and alpha-synuclein aggregation are each induced by PQ, but details of the cell death mechanisms involved remain unclear ... A Bak-dependent cell death mechanism /has been identified/ that is required for PQ-induced neurotoxicity. PQ induced morphological and biochemical features that were consistent with apoptosis, including dose-dependent cytochrome c release, with subsequent caspase-3 and poly(ADP-ribose) polymerase cleavage. Changes in nuclear morphology and loss of viability were blocked by cycloheximide, caspase inhibitor, and Bcl-2 overexpression. Evaluation of Bcl-2 family members showed that PQ induced high levels of Bak, Bid, BNip3, and Noxa. Small interfering RNA-mediated knockdown of BNip3, Noxa, and Bak each protected cells from PQ, but Bax knockdown did not. Finally, ... the sensitivity of Bak-deficient C57BL mice /was tested/ and found ... to be resistant to PQ treatments that depleted tyrosine hydroxylase immuno-positive neurons in the substantia nigra pars compacta of wild-type mice. For more Mechanism of Action (Complete) data for Paraquat (10 total), please visit the HSDB record page. |
---|---|
CAS No. |
1910-42-5 |
Molecular Formula |
C12H14ClN2+ |
Molecular Weight |
221.70 g/mol |
IUPAC Name |
1-methyl-4-(1-methylpyridin-1-ium-4-yl)pyridin-1-ium;chloride |
InChI |
InChI=1S/C12H14N2.ClH/c1-13-7-3-11(4-8-13)12-5-9-14(2)10-6-12;/h3-10H,1-2H3;1H/q+2;/p-1 |
InChI Key |
PHBHFYCOBFRUKJ-UHFFFAOYSA-M |
impurities |
The only impurity permitted in paraquat is free 4,4'-bipyridyl at a maximum level of 0.25%. |
SMILES |
C[N+]1=CC=C(C=C1)C2=CC=[N+](C=C2)C.[Cl-].[Cl-] |
Canonical SMILES |
C[N+]1=CC=C(C=C1)C2=CC=[N+](C=C2)C.[Cl-] |
Appearance |
Solid powder |
boiling_point |
Boiling point (760 mm Hg): Decomposes at 175-180 °C (347-356 °F) decomposes |
Color/Form |
Yellow solid Off-white powder ...a liquid in various strengths... The form marketed in the U.S. has a blue dye to keep it from being confused with beverages, a sharp odor to serve as a warning, and an agent to cause vomiting if someone drinks it. Yellow-white hygroscopic powde |
density |
1.24 |
melting_point |
Colorless crystals; mp: 300 °C (decomposes); very soluble in water, slightly soluble in lower alcohols. Insoluble in hydrocarbons. Hydrolyzed by alkali. Inactivated by inert clays and anionic surfactants; corrosive to metal; non-volatile /Paraquat dichloride/ A solid. MP: 300 °C (approximate decomposition) /Paraquat dimethyl sulfate/ 572 °F (decomposes) |
Key on ui other cas no. |
1910-42-5 |
physical_description |
Yellow, odorless solid; [HSDB] Technical grade is a dark red solution; [CHEMINFO] Commercially available as an aqueous solution with surfactants; [ACGIH] [herbicide] [Note: Paraquat may also be found commercially as a methyl sulfate |
Pictograms |
Acute Toxic; Irritant; Health Hazard; Environmental Hazard |
Purity |
>98% (or refer to the Certificate of Analysis) |
shelf_life |
>2 years if stored properly |
solubility |
In water, 6.2X10+5 mg/L at 20 °C Practically insoluble in organic solvents. |
storage |
Dry, dark and at 0 - 4 C for short term (days to weeks) or -20 C for long term (months to years). |
Synonyms |
Gramoxone Methyl Viologen Paragreen A Paraquat Viologen, Methyl |
vapor_pressure |
0.0000001 [mmHg] VP: Approximately 0 mm at 20 °C Vapor pressure of salts is very low, below 1X10-7 mm Hg /Paraquat salts/ <7.5X10-8 mm Hg at 25 °C <0.0000001 mmHg |
Origin of Product |
United States |
Synthesis routes and methods I
Procedure details
Synthesis routes and methods II
Procedure details
Retrosynthesis Analysis
AI-Powered Synthesis Planning: Our tool employs the Template_relevance Pistachio, Template_relevance Bkms_metabolic, Template_relevance Pistachio_ringbreaker, Template_relevance Reaxys, Template_relevance Reaxys_biocatalysis model, leveraging a vast database of chemical reactions to predict feasible synthetic routes.
One-Step Synthesis Focus: Specifically designed for one-step synthesis, it provides concise and direct routes for your target compounds, streamlining the synthesis process.
Accurate Predictions: Utilizing the extensive PISTACHIO, BKMS_METABOLIC, PISTACHIO_RINGBREAKER, REAXYS, REAXYS_BIOCATALYSIS database, our tool offers high-accuracy predictions, reflecting the latest in chemical research and data.
Strategy Settings
Precursor scoring | Relevance Heuristic |
---|---|
Min. plausibility | 0.01 |
Model | Template_relevance |
Template Set | Pistachio/Bkms_metabolic/Pistachio_ringbreaker/Reaxys/Reaxys_biocatalysis |
Top-N result to add to graph | 6 |
Feasible Synthetic Routes
Disclaimer and Information on In-Vitro Research Products
Please be aware that all articles and product information presented on BenchChem are intended solely for informational purposes. The products available for purchase on BenchChem are specifically designed for in-vitro studies, which are conducted outside of living organisms. In-vitro studies, derived from the Latin term "in glass," involve experiments performed in controlled laboratory settings using cells or tissues. It is important to note that these products are not categorized as medicines or drugs, and they have not received approval from the FDA for the prevention, treatment, or cure of any medical condition, ailment, or disease. We must emphasize that any form of bodily introduction of these products into humans or animals is strictly prohibited by law. It is essential to adhere to these guidelines to ensure compliance with legal and ethical standards in research and experimentation.