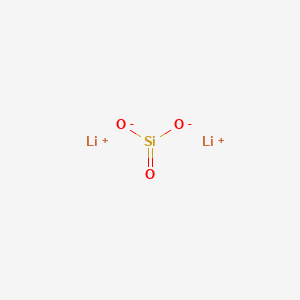
Lithium silicate
- Click on QUICK INQUIRY to receive a quote from our team of experts.
- With the quality product at a COMPETITIVE price, you can focus more on your research.
Overview
Description
Lithium silicate is a chemical compound composed of lithium, silicon, and oxygen. It is known for its high-temperature stability and is commonly used in various industrial applications, including ceramics, glass, and as a CO₂ capture material. The compound exists in different forms, such as lithium metasilicate (Li₂SiO₃) and lithium orthosilicate (Li₄SiO₄), each with unique properties and applications.
Mechanism of Action
Target of Action
Lithium metasilicate, also known as lithium silicate, is a compound that has been studied for its potential applications in various fields . .
Mode of Action
It is believed to inhibit enzymes that have magnesium as a co-factor . One such enzyme, the lithium-sensitive enzyme glycogen synthase kinase 3-beta (GSK3B), inhibits signaling induced by Brain-Derived Neurotrophic Factor (BDNF). Thus, lithium would be expected to enhance the activity of BDNF .
Biochemical Pathways
Lithium’s action on neurotransmitters and second messenger systems, as well as intracellular mechanisms of action which converge towards neuroprotection, have been studied . Lithium is known to decrease presynaptic dopamine activity and inactivate postsynaptic G protein, which reduces excitatory neurotransmission in the brain . It also enhances inhibitory neurotransmission by downregulating the NMDA receptor and inhibiting the myoinositol second messenger system directly .
Pharmacokinetics
The pharmacokinetics of lithium is linear within the dose regimen used in clinical practice. Lithium has an extremely narrow therapeutic window, which means that the concentrations required for therapeutic effects are close to concentrations that can cause toxicity .
Result of Action
It is known that lithium has neuroprotective effects . It is also believed to have anti-suicidal properties .
Action Environment
The action, efficacy, and stability of lithium metasilicate can be influenced by various environmental factors. For instance, the Li/Si molar ratio has a major effect on the size, morphology, and optical properties of lithium metasilicate nanomaterials . Furthermore, lithium metasilicate exhibits unique lattice symmetry (an orthorhombic crystal), valence and conduction bands, charge density distribution, and van Hove singularities .
Biochemical Analysis
Biochemical Properties
The critical multi-orbital hybridizations in Li–O and Si–O bonds, 2s– (2s, 2p, 2p, 2p) and (3s, 3p, 3p, 3p )– (2s, 2p, 2p, 2p ), respectively, have been identified .
Molecular Mechanism
Therefore, there exist many strong covalent bonds, with obvious anisotropy and non-uniformity .
Preparation Methods
Synthetic Routes and Reaction Conditions: Lithium silicate can be synthesized through several methods, including solid-state reactions, sol-gel processes, and hydrothermal synthesis. One common method involves mixing lithium carbonate (Li₂CO₃) with silicon dioxide (SiO₂) and heating the mixture to high temperatures (around 1000°C) to form this compound. Another method involves the reaction of lithium hydroxide (LiOH) with silicon dioxide in an aqueous solution, followed by drying and calcination.
Industrial Production Methods: In industrial settings, this compound is often produced using the solid-state reaction method due to its simplicity and scalability. The process involves the following steps:
- Mixing lithium carbonate and silicon dioxide in the desired stoichiometric ratio.
- Heating the mixture in a furnace at temperatures ranging from 800°C to 1000°C.
- Cooling the product and grinding it to the desired particle size.
Chemical Reactions Analysis
Types of Reactions: Lithium silicate undergoes various chemical reactions, including:
Oxidation: this compound can react with oxygen at high temperatures to form lithium oxide (Li₂O) and silicon dioxide.
Reduction: In the presence of reducing agents, this compound can be reduced to form lithium metal and silicon.
Substitution: this compound can undergo ion-exchange reactions with other metal ions, leading to the formation of different silicate compounds.
Common Reagents and Conditions:
Oxidation: Requires high temperatures (above 800°C) and an oxygen-rich environment.
Reduction: Typically involves the use of strong reducing agents such as hydrogen gas or carbon monoxide at elevated temperatures.
Substitution: Ion-exchange reactions can occur in aqueous solutions at room temperature or slightly elevated temperatures.
Major Products Formed:
Oxidation: Lithium oxide and silicon dioxide.
Reduction: Lithium metal and silicon.
Substitution: Various metal silicates depending on the substituting metal ion.
Scientific Research Applications
Lithium silicate has a wide range of scientific research applications, including:
Chemistry: Used as a precursor for the synthesis of other lithium compounds and as a catalyst in various chemical reactions.
Biology: Investigated for its potential use in biomedical applications, such as bone regeneration and dental materials.
Medicine: Explored for its use in drug delivery systems and as a component in bioactive glasses.
Industry: Widely used in the production of ceramics, glass, and as a high-temperature CO₂ capture material. It is also used in lithium-ion batteries as an anode material due to its high lithium-ion diffusivity and stability.
Comparison with Similar Compounds
Lithium silicate can be compared with other similar compounds, such as:
Sodium silicate (Na₂SiO₃): Similar in structure but has different chemical properties and applications. Sodium silicate is commonly used as a binder in cements and as a fireproofing agent.
Potassium silicate (K₂SiO₃): Used in agriculture as a fertilizer and in the production of specialty glasses.
Calcium silicate (Ca₂SiO₄): Widely used in construction materials, such as cement and insulation.
Uniqueness of this compound: this compound is unique due to its high lithium-ion diffusivity, making it an excellent material for lithium-ion batteries. Its high-temperature stability and ability to capture CO₂ efficiently also set it apart from other silicate compounds.
By understanding the properties, preparation methods, chemical reactions, and applications of this compound, researchers and industries can harness its potential for various innovative applications.
Properties
CAS No. |
10102-24-6 |
---|---|
Molecular Formula |
H2LiO3Si |
Molecular Weight |
85.1 g/mol |
IUPAC Name |
dilithium;dioxido(oxo)silane |
InChI |
InChI=1S/Li.H2O3Si/c;1-4(2)3/h;1-2H |
InChI Key |
YLICFGNKAGHNAH-UHFFFAOYSA-N |
SMILES |
[Li+].[Li+].[O-][Si](=O)[O-] |
Canonical SMILES |
[Li].O[Si](=O)O |
Key on ui other cas no. |
12627-14-4 63985-45-5 10102-24-6 |
Pictograms |
Corrosive; Irritant |
Origin of Product |
United States |
- Q: How can spectroscopic techniques be used to study the structure of lithium silicate?
- A: Raman spectroscopy has been instrumental in understanding the structure of this compound glasses and melts at high temperatures []. This technique reveals information about the structural units present, including those with bridging and non-bridging oxygen atoms, providing valuable insights into the melt's behavior and properties.
- A: Adding this compound to LiNi0.8Co0.1Mn0.1O2 cathode materials improves their cycle performance and rate capability [, ]. This compound, while not incorporated into the crystal lattice, localizes at grain boundaries and pores, strengthening the material and inhibiting crack formation, leading to improved battery longevity.
- A: While promising, lithium silicates face challenges like moderate capture kinetics and limited stability over multiple cycles. To overcome these, researchers have explored synthesizing this compound nanosheets (LSNs), which offer a high surface area for CO2 adsorption, enhancing both capture capacity and kinetics []. The nanosheet morphology also mitigates the formation of a thick carbonate shell during cycling, improving stability.
A: Lithium silicates, specifically lithium orthosilicate (Li4SiO4), are promising materials for high-temperature CO2 capture due to their high theoretical capacity (8 mmol g-1) [, , , , , , ]. They react with CO2 at high temperatures (around 700°C), forming lithium carbonate and a secondary this compound phase. The process can be reversed at even higher temperatures (around 850°C), releasing the captured CO2 and regenerating the sorbent. This reversible reaction makes lithium silicates suitable for continuous CO2 capture systems using thermal swing processes.
- Q: How is density functional theory (DFT) being used to understand lithium silicates?
- A: DFT calculations have provided valuable insights into the electronic structures and mechanical properties of lithium silicates like Li₂SiO₃ and Li4SiO4 []. These calculations help predict band gaps, electron distributions, and bonding characteristics, aiding in the design and optimization of these materials for applications like tritium breeding materials in fusion reactors.
- Q: What can molecular dynamics (MD) simulations tell us about this compound liquids?
- A: MD simulations have been employed to investigate the behavior of this compound liquids under compression []. These simulations shed light on the microstructure, revealing a transition from low to high coordination of atoms with increasing pressure. Additionally, the simulations provide information on the dynamics of these liquids, including diffusion coefficients, offering a deeper understanding of their properties under extreme conditions.
- A: Researchers are exploring incorporating other elements, like germanium, into this compound composites to improve CO2 capture kinetics at lower temperatures []. These composites have shown promising results in enhancing the absorption rate and capacity, expanding the potential applications of this compound-based sorbents.
- A: Preliminary studies have demonstrated the possibility of synthesizing lithium metasilicate from waste container glass through hydrothermal reactions with lithium hydroxide solutions []. This approach holds potential for recycling waste glass while producing valuable materials, contributing to a circular economy and resource conservation.
Disclaimer and Information on In-Vitro Research Products
Please be aware that all articles and product information presented on BenchChem are intended solely for informational purposes. The products available for purchase on BenchChem are specifically designed for in-vitro studies, which are conducted outside of living organisms. In-vitro studies, derived from the Latin term "in glass," involve experiments performed in controlled laboratory settings using cells or tissues. It is important to note that these products are not categorized as medicines or drugs, and they have not received approval from the FDA for the prevention, treatment, or cure of any medical condition, ailment, or disease. We must emphasize that any form of bodily introduction of these products into humans or animals is strictly prohibited by law. It is essential to adhere to these guidelines to ensure compliance with legal and ethical standards in research and experimentation.