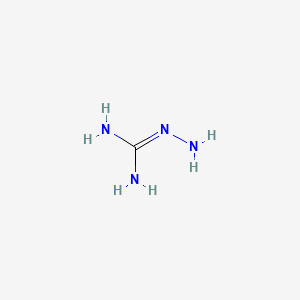
Aminoguanidine
Overview
Description
Pimagedine, also known as aminoguanidine, is a small organic compound with the chemical formula CH6N4. It has been investigated primarily for its potential therapeutic effects in managing diabetic nephropathy. Pimagedine functions as an inhibitor of diamine oxidase and nitric oxide synthase, and it acts to reduce levels of advanced glycation end products (AGEs) by interacting with reactive dicarbonyl compounds such as 3-deoxyglucosone, glyoxal, and methylglyoxal .
Preparation Methods
Synthetic Routes and Reaction Conditions
Pimagedine can be synthesized through several methods. One common synthetic route involves the reaction of hydrazine with cyanamide under controlled conditions. The reaction typically proceeds as follows:
- Hydrazine reacts with cyanamide to form aminoguanidine.
- The reaction is carried out in an aqueous medium at a temperature of around 50-60°C.
- The product is then purified through recrystallization.
Industrial Production Methods
Industrial production of pimagedine follows similar synthetic routes but on a larger scale. The process involves:
- Large-scale reaction vessels to accommodate the reactants.
- Continuous monitoring of reaction conditions to ensure optimal yield.
- Use of advanced purification techniques such as chromatography to obtain high-purity pimagedine.
Chemical Reactions Analysis
Types of Reactions
Pimagedine undergoes several types of chemical reactions, including:
Oxidation: Pimagedine can be oxidized to form various oxidation products.
Reduction: It can also undergo reduction reactions under specific conditions.
Substitution: Pimagedine can participate in substitution reactions where one of its functional groups is replaced by another group.
Common Reagents and Conditions
Oxidation: Common oxidizing agents include hydrogen peroxide and potassium permanganate.
Reduction: Reducing agents such as sodium borohydride can be used.
Substitution: Various halogenating agents and nucleophiles are employed in substitution reactions.
Major Products Formed
Oxidation: Oxidation of pimagedine can lead to the formation of guanidine derivatives.
Reduction: Reduction reactions typically yield aminoguanidine derivatives.
Substitution: Substitution reactions can produce a wide range of substituted guanidine compounds.
Scientific Research Applications
Chemistry: Used as a reagent in organic synthesis and as an intermediate in the production of other compounds.
Biology: Investigated for its role in inhibiting the formation of AGEs, which are implicated in various age-related diseases.
Medicine: Explored for its therapeutic potential in managing diabetic nephropathy and other complications of diabetes.
Industry: Utilized in the production of pharmaceuticals and as a stabilizer in certain industrial processes
Mechanism of Action
Pimagedine exerts its effects primarily by inhibiting the formation of glycosylated proteins (AGEs). It achieves this by:
Inhibiting Aldose Reductase: This enzyme is involved in the polyol pathway, which contributes to the formation of AGEs.
Inhibiting Diamine Oxidase and Nitric Oxide Synthase: These enzymes are involved in the production of reactive oxygen species and nitric oxide, respectively
Comparison with Similar Compounds
Pimagedine is often compared with other AGE inhibitors such as:
Alagebrium: Unlike pimagedine, alagebrium breaks existing AGE cross-links.
Benfotiamine: This compound works by blocking multiple pathways that lead to AGE formation.
Pyridoxamine: Inhibits the formation of AGEs by scavenging reactive carbonyl intermediates.
Pimagedine is unique in its dual inhibition of diamine oxidase and nitric oxide synthase, which sets it apart from other AGE inhibitors .
Properties
IUPAC Name |
2-aminoguanidine | |
---|---|---|
Source | PubChem | |
URL | https://pubchem.ncbi.nlm.nih.gov | |
Description | Data deposited in or computed by PubChem | |
InChI |
InChI=1S/CH6N4/c2-1(3)5-4/h4H2,(H4,2,3,5) | |
Source | PubChem | |
URL | https://pubchem.ncbi.nlm.nih.gov | |
Description | Data deposited in or computed by PubChem | |
InChI Key |
HAMNKKUPIHEESI-UHFFFAOYSA-N | |
Source | PubChem | |
URL | https://pubchem.ncbi.nlm.nih.gov | |
Description | Data deposited in or computed by PubChem | |
Canonical SMILES |
C(=NN)(N)N | |
Source | PubChem | |
URL | https://pubchem.ncbi.nlm.nih.gov | |
Description | Data deposited in or computed by PubChem | |
Molecular Formula |
CH6N4 | |
Source | PubChem | |
URL | https://pubchem.ncbi.nlm.nih.gov | |
Description | Data deposited in or computed by PubChem | |
Related CAS |
1937-19-5 (hydrochloride) | |
Record name | Pimagedine [INN] | |
Source | ChemIDplus | |
URL | https://pubchem.ncbi.nlm.nih.gov/substance/?source=chemidplus&sourceid=0000079174 | |
Description | ChemIDplus is a free, web search system that provides access to the structure and nomenclature authority files used for the identification of chemical substances cited in National Library of Medicine (NLM) databases, including the TOXNET system. | |
DSSTOX Substance ID |
DTXSID5040964 | |
Record name | Aminoguanidine | |
Source | EPA DSSTox | |
URL | https://comptox.epa.gov/dashboard/DTXSID5040964 | |
Description | DSSTox provides a high quality public chemistry resource for supporting improved predictive toxicology. | |
Molecular Weight |
74.09 g/mol | |
Source | PubChem | |
URL | https://pubchem.ncbi.nlm.nih.gov | |
Description | Data deposited in or computed by PubChem | |
Mechanism of Action |
Pimagedine reportedly inhibits the formation of glycosylated proteins (advanced glycosylation end-products) and has other actions including inhibition of aldose reductase. | |
Record name | Pimagedine | |
Source | DrugBank | |
URL | https://www.drugbank.ca/drugs/DB05383 | |
Description | The DrugBank database is a unique bioinformatics and cheminformatics resource that combines detailed drug (i.e. chemical, pharmacological and pharmaceutical) data with comprehensive drug target (i.e. sequence, structure, and pathway) information. | |
Explanation | Creative Common's Attribution-NonCommercial 4.0 International License (http://creativecommons.org/licenses/by-nc/4.0/legalcode) | |
CAS No. |
79-17-4 | |
Record name | Aminoguanidine | |
Source | CAS Common Chemistry | |
URL | https://commonchemistry.cas.org/detail?cas_rn=79-17-4 | |
Description | CAS Common Chemistry is an open community resource for accessing chemical information. Nearly 500,000 chemical substances from CAS REGISTRY cover areas of community interest, including common and frequently regulated chemicals, and those relevant to high school and undergraduate chemistry classes. This chemical information, curated by our expert scientists, is provided in alignment with our mission as a division of the American Chemical Society. | |
Explanation | The data from CAS Common Chemistry is provided under a CC-BY-NC 4.0 license, unless otherwise stated. | |
Record name | Pimagedine [INN] | |
Source | ChemIDplus | |
URL | https://pubchem.ncbi.nlm.nih.gov/substance/?source=chemidplus&sourceid=0000079174 | |
Description | ChemIDplus is a free, web search system that provides access to the structure and nomenclature authority files used for the identification of chemical substances cited in National Library of Medicine (NLM) databases, including the TOXNET system. | |
Record name | Pimagedine | |
Source | DrugBank | |
URL | https://www.drugbank.ca/drugs/DB05383 | |
Description | The DrugBank database is a unique bioinformatics and cheminformatics resource that combines detailed drug (i.e. chemical, pharmacological and pharmaceutical) data with comprehensive drug target (i.e. sequence, structure, and pathway) information. | |
Explanation | Creative Common's Attribution-NonCommercial 4.0 International License (http://creativecommons.org/licenses/by-nc/4.0/legalcode) | |
Record name | Aminoguanidine | |
Source | EPA DSSTox | |
URL | https://comptox.epa.gov/dashboard/DTXSID5040964 | |
Description | DSSTox provides a high quality public chemistry resource for supporting improved predictive toxicology. | |
Record name | Aminoguanidine | |
Source | European Chemicals Agency (ECHA) | |
URL | https://echa.europa.eu/substance-information/-/substanceinfo/100.001.076 | |
Description | The European Chemicals Agency (ECHA) is an agency of the European Union which is the driving force among regulatory authorities in implementing the EU's groundbreaking chemicals legislation for the benefit of human health and the environment as well as for innovation and competitiveness. | |
Explanation | Use of the information, documents and data from the ECHA website is subject to the terms and conditions of this Legal Notice, and subject to other binding limitations provided for under applicable law, the information, documents and data made available on the ECHA website may be reproduced, distributed and/or used, totally or in part, for non-commercial purposes provided that ECHA is acknowledged as the source: "Source: European Chemicals Agency, http://echa.europa.eu/". Such acknowledgement must be included in each copy of the material. ECHA permits and encourages organisations and individuals to create links to the ECHA website under the following cumulative conditions: Links can only be made to webpages that provide a link to the Legal Notice page. | |
Record name | PIMAGEDINE | |
Source | FDA Global Substance Registration System (GSRS) | |
URL | https://gsrs.ncats.nih.gov/ginas/app/beta/substances/SCQ4EZQ113 | |
Description | The FDA Global Substance Registration System (GSRS) enables the efficient and accurate exchange of information on what substances are in regulated products. Instead of relying on names, which vary across regulatory domains, countries, and regions, the GSRS knowledge base makes it possible for substances to be defined by standardized, scientific descriptions. | |
Explanation | Unless otherwise noted, the contents of the FDA website (www.fda.gov), both text and graphics, are not copyrighted. They are in the public domain and may be republished, reprinted and otherwise used freely by anyone without the need to obtain permission from FDA. Credit to the U.S. Food and Drug Administration as the source is appreciated but not required. | |
Retrosynthesis Analysis
AI-Powered Synthesis Planning: Our tool employs the Template_relevance Pistachio, Template_relevance Bkms_metabolic, Template_relevance Pistachio_ringbreaker, Template_relevance Reaxys, Template_relevance Reaxys_biocatalysis model, leveraging a vast database of chemical reactions to predict feasible synthetic routes.
One-Step Synthesis Focus: Specifically designed for one-step synthesis, it provides concise and direct routes for your target compounds, streamlining the synthesis process.
Accurate Predictions: Utilizing the extensive PISTACHIO, BKMS_METABOLIC, PISTACHIO_RINGBREAKER, REAXYS, REAXYS_BIOCATALYSIS database, our tool offers high-accuracy predictions, reflecting the latest in chemical research and data.
Strategy Settings
Precursor scoring | Relevance Heuristic |
---|---|
Min. plausibility | 0.01 |
Model | Template_relevance |
Template Set | Pistachio/Bkms_metabolic/Pistachio_ringbreaker/Reaxys/Reaxys_biocatalysis |
Top-N result to add to graph | 6 |
Feasible Synthetic Routes
Disclaimer and Information on In-Vitro Research Products
Please be aware that all articles and product information presented on BenchChem are intended solely for informational purposes. The products available for purchase on BenchChem are specifically designed for in-vitro studies, which are conducted outside of living organisms. In-vitro studies, derived from the Latin term "in glass," involve experiments performed in controlled laboratory settings using cells or tissues. It is important to note that these products are not categorized as medicines or drugs, and they have not received approval from the FDA for the prevention, treatment, or cure of any medical condition, ailment, or disease. We must emphasize that any form of bodily introduction of these products into humans or animals is strictly prohibited by law. It is essential to adhere to these guidelines to ensure compliance with legal and ethical standards in research and experimentation.