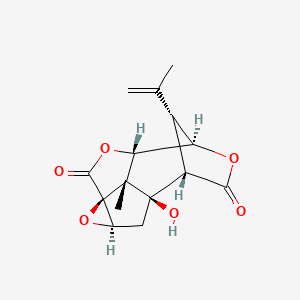
Picrotoxinin
Overview
Description
Picrotoxinin is a naturally occurring compound found in the seeds of the Anamirta cocculus plant. It is a part of the picrotoxin complex, which also includes picrotin. This compound is known for its potent convulsant properties and its ability to act as a noncompetitive antagonist of gamma-aminobutyric acid (GABA) receptors .
Mechanism of Action
Target of Action
Picrotoxinin primarily targets the GABA A receptors . These receptors are ligand-gated ion channels chiefly concerned with the passing of chloride ions across the cell membrane . They play a crucial role in inhibitory neurotransmission in the central nervous system .
Mode of Action
This compound acts as a noncompetitive antagonist at GABA A receptors . It directly antagonizes the GABA A receptor channel, preventing the permeability of chloride ions across the cell membrane . This action promotes an inhibitory influence on the target neuron .
Biochemical Pathways
The primary biochemical pathway affected by this compound is the GABAergic pathway . By blocking the GABA A receptors, this compound disrupts the normal inhibitory function of GABA (gamma-aminobutyric acid), leading to increased neuronal excitability .
Pharmacokinetics
This compound, the active component of Picrotoxin, hydrolyses quickly into picrotoxic acid . It has a short in vivo half-life and is moderately brain penetrant (brain/plasma ratio 0.3) . These properties impact its bioavailability and distribution within the body .
Result of Action
The molecular and cellular effects of this compound’s action primarily manifest as stimulation and convulsion . By antagonizing the GABA A receptors, this compound disrupts the normal inhibitory neurotransmission, leading to increased neuronal excitability . This can result in seizures and, in high enough doses, respiratory paralysis .
Biochemical Analysis
Biochemical Properties
Picrotoxinin plays a significant role in biochemical reactions by interacting with various enzymes, proteins, and other biomolecules. It primarily targets GABA-A receptors, which are ligand-gated ion channels responsible for mediating inhibitory neurotransmission in the brain. By binding to these receptors, this compound blocks the chloride ionophore, preventing chloride ions from passing through the channel. This inhibition reduces the inhibitory effects of GABA, leading to increased neuronal excitability .
Cellular Effects
This compound exerts profound effects on various types of cells and cellular processes. In neurons, it disrupts normal inhibitory signaling by blocking GABA-A receptors, leading to increased neuronal firing and excitability. This can result in convulsions and seizures. Additionally, this compound influences cell signaling pathways, gene expression, and cellular metabolism by altering the balance of excitatory and inhibitory signals in the brain .
Molecular Mechanism
At the molecular level, this compound exerts its effects by binding to the GABA-A receptor chloride channel. This binding prevents the flow of chloride ions, which are essential for the inhibitory action of GABA. As a result, this compound reduces the conductance through the channel by decreasing both the opening frequency and the mean open time. This leads to a reduction in the inhibitory influence on target neurons, promoting excitability and convulsant activity .
Temporal Effects in Laboratory Settings
In laboratory settings, the effects of this compound can vary over time. This compound is known to hydrolyze quickly into picrotoxic acid, which has a shorter half-life in vivo. This rapid degradation can influence the stability and long-term effects of this compound on cellular function. Studies have shown that this compound can significantly shorten the period of circadian oscillations in gene expression, indicating its impact on cellular rhythms and stability .
Dosage Effects in Animal Models
The effects of this compound vary with different dosages in animal models. At low doses, this compound can induce mild convulsions, while higher doses can lead to severe seizures and even death. The threshold for toxic effects is relatively low, with lethal doses reported to be as low as 0.357 mg/kg in some studies. The compound’s convulsant properties make it a valuable tool for studying seizure mechanisms and testing anticonvulsant drugs .
Metabolic Pathways
This compound is metabolized in the body through hydrolysis into picrotoxic acid. This metabolic pathway involves the action of liver enzymes, which break down this compound into its less active form. The rapid hydrolysis of this compound contributes to its short half-life and limited duration of action in vivo. Understanding these metabolic pathways is crucial for developing strategies to mitigate its toxic effects .
Transport and Distribution
This compound is moderately brain-penetrant, with a brain/plasma ratio of approximately 0.3. This indicates that a significant portion of the compound can cross the blood-brain barrier and exert its effects on the central nervous system. The distribution of this compound within cells and tissues is influenced by its interactions with plasma proteins and transporters, which can affect its localization and accumulation .
Subcellular Localization
The subcellular localization of this compound is primarily associated with its target, the GABA-A receptor chloride channel. By binding to these receptors, this compound exerts its inhibitory effects on chloride ion permeability. This localization is critical for its convulsant activity, as it directly impacts the function of inhibitory neurotransmission in neurons .
Preparation Methods
Picrotoxinin can be isolated from the seeds of Anamirta cocculus through a series of extraction and purification steps. Additionally, several synthetic routes have been developed to produce this compound. One notable method involves the use of carvone as a stereochemical template. This synthesis includes steps such as Claisen rearrangement, organoselenium-mediated reduction of an epoxy ketone, and stereospecific construction of a glycidic ester .
Chemical Reactions Analysis
Picrotoxinin undergoes various chemical reactions, including oxidation, reduction, and substitution reactions. Common reagents used in these reactions include trifluoroacetic anhydride in pyridine for protection of the cis alcohol group . The major products formed from these reactions depend on the specific conditions and reagents used.
Scientific Research Applications
Picrotoxinin is extensively used in scientific research due to its ability to antagonize GABA receptors. It is employed as a research tool to study the function of GABA in the central nervous system. Additionally, this compound has been used as a central nervous system stimulant and an antidote for poisoning by central nervous system depressants, especially barbiturates . Its applications extend to the fields of chemistry, biology, medicine, and industry.
Comparison with Similar Compounds
Picrotoxinin is often compared with other GABA receptor antagonists such as bicuculline and gabazine. While bicuculline and gabazine are competitive antagonists of GABA A receptors, this compound acts as a noncompetitive antagonist . This distinction highlights the uniqueness of this compound in its mode of action. Other similar compounds include tetramethylenedisulfotetramine (TETS), which also inhibits GABA A receptors but is significantly more lethal than this compound .
Properties
CAS No. |
17617-45-7 |
---|---|
Molecular Formula |
C15H16O6 |
Molecular Weight |
292.28 g/mol |
IUPAC Name |
(1S,3R,5R,8S,9R,12S,13S,14R)-1-hydroxy-13-methyl-14-prop-1-en-2-yl-4,7,10-trioxapentacyclo[6.4.1.19,12.03,5.05,13]tetradecane-6,11-dione |
InChI |
InChI=1S/C15H16O6/c1-5(2)7-8-11(16)19-9(7)10-13(3)14(8,18)4-6-15(13,21-6)12(17)20-10/h6-10,18H,1,4H2,2-3H3/t6-,7+,8-,9-,10-,13+,14+,15-/m1/s1 |
InChI Key |
PIMZUZSSNYHVCU-CKZDGMHMSA-N |
SMILES |
CC(=C)C1C2C3C4(C(C1C(=O)O2)(CC5C4(O5)C(=O)O3)O)C |
Isomeric SMILES |
CC(=C)[C@@H]1[C@@H]2[C@@H]3[C@]4([C@@]([C@H]1C(=O)O2)(C[C@@H]5[C@@]4(O5)C(=O)O3)O)C |
Canonical SMILES |
CC(=C)C1C2C3C4(C(C1C(=O)O2)(CC5C4(O5)C(=O)O3)O)C |
Appearance |
Solid powder |
17617-45-7 | |
Pictograms |
Acute Toxic |
Purity |
>98% (or refer to the Certificate of Analysis) |
shelf_life |
>3 years if stored properly |
solubility |
Soluble in DMSO |
storage |
Dry, dark and at 0 - 4 C for short term (days to weeks) or -20 C for long term (months to years). |
Synonyms |
Picrotoxinin; NSC 129537; NSC-129537; NSC129537 |
Origin of Product |
United States |
Retrosynthesis Analysis
AI-Powered Synthesis Planning: Our tool employs the Template_relevance Pistachio, Template_relevance Bkms_metabolic, Template_relevance Pistachio_ringbreaker, Template_relevance Reaxys, Template_relevance Reaxys_biocatalysis model, leveraging a vast database of chemical reactions to predict feasible synthetic routes.
One-Step Synthesis Focus: Specifically designed for one-step synthesis, it provides concise and direct routes for your target compounds, streamlining the synthesis process.
Accurate Predictions: Utilizing the extensive PISTACHIO, BKMS_METABOLIC, PISTACHIO_RINGBREAKER, REAXYS, REAXYS_BIOCATALYSIS database, our tool offers high-accuracy predictions, reflecting the latest in chemical research and data.
Strategy Settings
Precursor scoring | Relevance Heuristic |
---|---|
Min. plausibility | 0.01 |
Model | Template_relevance |
Template Set | Pistachio/Bkms_metabolic/Pistachio_ringbreaker/Reaxys/Reaxys_biocatalysis |
Top-N result to add to graph | 6 |
Feasible Synthetic Routes
Q1: What is the primary target of picrotoxinin?
A1: this compound acts as a non-competitive antagonist of the γ-aminobutyric acid type A receptors (GABAARs) [[8]]. GABAARs are the primary mediators of inhibitory neurotransmission in the nervous system [[29]].
Q2: How does this compound affect GABAARs?
A2: Unlike competitive antagonists that bind at the GABA recognition site, this compound binds within the ion channel pore [[29]]. This binding physically obstructs chloride ion flow through the channel, preventing GABA-induced neuronal inhibition [[8], []].
Q3: What are the downstream effects of this compound binding to GABAARs?
A3: By blocking the inhibitory action of GABA, this compound leads to excessive neuronal excitation [[24]]. This can manifest as convulsions and seizures in living organisms [[8], []].
Q4: What is the molecular formula and weight of this compound?
A4: While not explicitly stated in the provided abstracts, the molecular formula of this compound is C15H16O6, and its molecular weight is 292.29 g/mol.
Q5: Is there any spectroscopic data available for this compound?
A5: The provided research articles focus on the pharmacological aspects and do not delve into detailed spectroscopic characterization of this compound.
Q6: How does the presence of a hydroxyl group at the 6-position of picrotoxane compounds affect their activity?
A6: The hydroxyl group at the 6-position appears to be crucial for antagonizing the effects of GABA but not β-alanine or taurine in the frog spinal cord [[1]]. It is also important for blocking presynaptic inhibition [[1]].
Q7: Does modifying proline 309 in the human ρ1 subunit impact this compound sensitivity?
A7: Substituting proline 309 with serine (the corresponding amino acid in the human ρ2 subunit) decreases the this compound sensitivity of the ρ1 subunit by four-fold [[10], []]. This suggests that the amino acid at position 309 plays a significant role in this compound binding.
Q8: Are there known mechanisms of resistance to this compound in insects?
A8: Yes, cyclodiene-resistant insects, such as certain strains of Drosophila melanogaster, exhibit cross-resistance to this compound [[4], [], [], []]. This resistance is attributed to mutations in the GABAAR gene, specifically at the this compound binding site [[16], [], []].
Q9: Does the A302→S mutation in the Drosophila RDL GABA receptor affect fipronil sensitivity?
A9: Yes, this mutation, which confers resistance to dieldrin and this compound, also reduces the sensitivity of RDL receptors to fipronil [[28]]. This suggests a shared binding site or mechanism of action for these insecticides.
Q10: What is the effect of this compound on crayfish muscle?
A10: [3H]α-Dihydrothis compound binds to high-affinity sites in crayfish muscle homogenates, particularly in sarcolemma fractions [[3]]. These binding sites are thought to be related to the regulation of inhibitory chloride ionophores, potentially at a site distinct from the GABA receptor [[3]].
Q11: How do pyrazolopyridines, like etazolate, interact with this compound binding sites?
A11: Pyrazolopyridines enhance [3H]diazepam binding to benzodiazepine receptors, and this enhancement is blocked by this compound [[19], []]. These findings suggest an interaction between pyrazolopyridines, benzodiazepine, and this compound binding sites within the GABAAR complex [[19], []].
Q12: Can this compound be used to differentiate between GABA and glycine receptors?
A12: While this compound is considered a GABAA receptor antagonist, it can also inhibit glycine receptors, although with lower potency [[26], []]. This overlap in activity emphasizes the importance of careful experimental design and interpretation when using this compound to study these receptor systems.
Q13: What radioligands are commonly used to study this compound binding sites?
A13: Researchers utilize radiolabeled ligands like [3H]α-dihydrothis compound and [35S]t-butylbicyclophosphorothionate ([35S]TBPS) to investigate this compound binding sites in the brain [[5], [], [], [], [], [], []].
Q14: What techniques are used to study the effects of this compound on neuronal activity?
A14: Electrophysiological techniques, such as patch-clamp recordings, are employed to investigate the effects of this compound on neuronal activity at the single-channel level [[2], [], [], []]. These methods provide valuable insights into the kinetics of this compound blockade on GABAARs.
Q15: Is this compound considered toxic?
A15: Yes, this compound is a potent convulsant [[4], [], [], []]. It exerts its toxicity by antagonizing GABAARs, leading to uncontrolled neuronal excitation [[8], []]. The provided research focuses on the mechanism of action and pharmacological characterization rather than detailed toxicological profiles.
Disclaimer and Information on In-Vitro Research Products
Please be aware that all articles and product information presented on BenchChem are intended solely for informational purposes. The products available for purchase on BenchChem are specifically designed for in-vitro studies, which are conducted outside of living organisms. In-vitro studies, derived from the Latin term "in glass," involve experiments performed in controlled laboratory settings using cells or tissues. It is important to note that these products are not categorized as medicines or drugs, and they have not received approval from the FDA for the prevention, treatment, or cure of any medical condition, ailment, or disease. We must emphasize that any form of bodily introduction of these products into humans or animals is strictly prohibited by law. It is essential to adhere to these guidelines to ensure compliance with legal and ethical standards in research and experimentation.