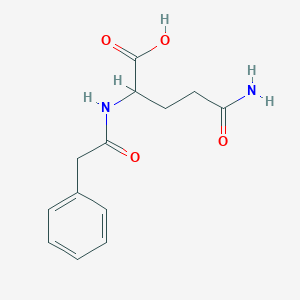
Phenylacetylglutamine
Overview
Description
Phenylacetylglutamine is a primary human metabolite formed from phenylacetate in the presence of glutamine in the liver . It is also considered a gut-microbiome derived uremic toxin . Phenylacetylglutamine urine levels serve as an effective biomarker for the excretion of nitrogenous waste .
Molecular Structure Analysis
Phenylacetylglutamine has a molecular formula of C13H16N2O4 . Its average mass is 264.277 Da and its monoisotopic mass is 264.110992 Da .Physical And Chemical Properties Analysis
Phenylacetylglutamine has a molecular formula of C13H16N2O4 . Its molecular weight is 264.28 . The CAS Registry Number for Phenylacetylglutamine is 28047-15-6 .Scientific Research Applications
Cardiovascular Diseases
Phenylacetylglutamine (PAGln) has been linked to cardiovascular diseases (CVDs). It is a metabolite derived from phenylalanine metabolism, which involves the gut microbiota. Studies suggest that PAGln levels are associated with the risk of various CVDs. The metabolic pathways of PAGln production and quantitative measurement methods are areas of active research .
Gut Microbiota Interactions
The gut microbiota plays a crucial role in the metabolism of phenylalanine, leading to the production of PAGln. Disordered gut microbiota has been implicated in the progression of atrial fibrillation (AF), and PAGln is being studied for its potential relationship with AF and other gut microbiota-related conditions .
Future Directions
The gut microbiome, including Phenylacetylglutamine, is a beacon of hope for improving the management of cardiometabolic disease in the age of precision medicine . Available studies point to the potential of gut microbiome-based therapeutics, which remains to be tested in appropriately designed clinical trials . Further preclinical research is essential to provide answers to the existing obstacles, with the ultimate goal of enhancing patient care .
Mechanism of Action
Target of Action
Phenylacetylglutamine (PAGln) is a gut microbiota-derived metabolite that is clinically and mechanistically linked to cardiovascular disease (CVD) . The primary targets of PAGln are endothelial cells , where it induces oxidative stress via a NOX2-dependent pathway .
Mode of Action
PAGln interacts with its targets, the endothelial cells, by inducing oxidative stress . This interaction results in an upregulation of NOX2 and NF-kB protein levels, while NOX4 levels remain unchanged .
Biochemical Pathways
The microbiota-dependent metabolism of phenylalanine produces phenylacetic acid (PAA), which is a crucial intermediate that is catalyzed by diverse microbial catalytic pathways . PAA conjugates with glutamine and glycine in the liver and kidney to predominantly form phenylacetylglutamine in humans and phenylacetylglycine in rodents .
Pharmacokinetics
Phenylacetic acid (PAA) and phenylacetylglutamine (PAGN) pharmacokinetic data from clinical studies indicate that PAA exhibits nonlinear pharmacokinetics . PAA exposure was found to be 35% higher in Child–Pugh C than in Child–Pugh B . PAGN renal clearance decreased by five-fold in severe renal impairment compared with subjects with normal renal function .
Result of Action
PAGln treatment for 72 hours significantly increased the fluorescence intensity after CellROX Green staining . This suggests that PAGln treatment leads to an increase in reactive oxygen species (ROS) formation . This oxidative stress could potentially lead to various cellular effects, including damage to cell structures like lipids, proteins, and DNA.
Action Environment
Environmental factors such as diet, lifestyle, and the presence of other diseases can influence the action of PAGln . For instance, dietary protein intake and the abundance of PAA-producing Proteobacteria can cause elevated PAGln levels . Furthermore, the presence of diseases like cardiovascular disease can also influence the action and efficacy of PAGln .
properties
IUPAC Name |
(2S)-5-amino-5-oxo-2-[(2-phenylacetyl)amino]pentanoic acid | |
---|---|---|
Source | PubChem | |
URL | https://pubchem.ncbi.nlm.nih.gov | |
Description | Data deposited in or computed by PubChem | |
InChI |
InChI=1S/C13H16N2O4/c14-11(16)7-6-10(13(18)19)15-12(17)8-9-4-2-1-3-5-9/h1-5,10H,6-8H2,(H2,14,16)(H,15,17)(H,18,19)/t10-/m0/s1 | |
Source | PubChem | |
URL | https://pubchem.ncbi.nlm.nih.gov | |
Description | Data deposited in or computed by PubChem | |
InChI Key |
JFLIEFSWGNOPJJ-JTQLQIEISA-N | |
Source | PubChem | |
URL | https://pubchem.ncbi.nlm.nih.gov | |
Description | Data deposited in or computed by PubChem | |
Canonical SMILES |
C1=CC=C(C=C1)CC(=O)NC(CCC(=O)N)C(=O)O | |
Source | PubChem | |
URL | https://pubchem.ncbi.nlm.nih.gov | |
Description | Data deposited in or computed by PubChem | |
Isomeric SMILES |
C1=CC=C(C=C1)CC(=O)N[C@@H](CCC(=O)N)C(=O)O | |
Source | PubChem | |
URL | https://pubchem.ncbi.nlm.nih.gov | |
Description | Data deposited in or computed by PubChem | |
Molecular Formula |
C13H16N2O4 | |
Source | PubChem | |
URL | https://pubchem.ncbi.nlm.nih.gov | |
Description | Data deposited in or computed by PubChem | |
DSSTOX Substance ID |
DTXSID90182324 | |
Record name | Phenylacetylglutamine | |
Source | EPA DSSTox | |
URL | https://comptox.epa.gov/dashboard/DTXSID90182324 | |
Description | DSSTox provides a high quality public chemistry resource for supporting improved predictive toxicology. | |
Molecular Weight |
264.28 g/mol | |
Source | PubChem | |
URL | https://pubchem.ncbi.nlm.nih.gov | |
Description | Data deposited in or computed by PubChem | |
Physical Description |
Solid | |
Record name | Phenylacetylglutamine | |
Source | Human Metabolome Database (HMDB) | |
URL | http://www.hmdb.ca/metabolites/HMDB0006344 | |
Description | The Human Metabolome Database (HMDB) is a freely available electronic database containing detailed information about small molecule metabolites found in the human body. | |
Explanation | HMDB is offered to the public as a freely available resource. Use and re-distribution of the data, in whole or in part, for commercial purposes requires explicit permission of the authors and explicit acknowledgment of the source material (HMDB) and the original publication (see the HMDB citing page). We ask that users who download significant portions of the database cite the HMDB paper in any resulting publications. | |
Product Name |
Phenylacetylglutamine | |
CAS RN |
28047-15-6 | |
Record name | Phenylacetylglutamine | |
Source | CAS Common Chemistry | |
URL | https://commonchemistry.cas.org/detail?cas_rn=28047-15-6 | |
Description | CAS Common Chemistry is an open community resource for accessing chemical information. Nearly 500,000 chemical substances from CAS REGISTRY cover areas of community interest, including common and frequently regulated chemicals, and those relevant to high school and undergraduate chemistry classes. This chemical information, curated by our expert scientists, is provided in alignment with our mission as a division of the American Chemical Society. | |
Explanation | The data from CAS Common Chemistry is provided under a CC-BY-NC 4.0 license, unless otherwise stated. | |
Record name | Phenylacetylglutamine | |
Source | ChemIDplus | |
URL | https://pubchem.ncbi.nlm.nih.gov/substance/?source=chemidplus&sourceid=0028047156 | |
Description | ChemIDplus is a free, web search system that provides access to the structure and nomenclature authority files used for the identification of chemical substances cited in National Library of Medicine (NLM) databases, including the TOXNET system. | |
Record name | Phenylacetylglutamine | |
Source | DrugBank | |
URL | https://www.drugbank.ca/drugs/DB17167 | |
Description | The DrugBank database is a unique bioinformatics and cheminformatics resource that combines detailed drug (i.e. chemical, pharmacological and pharmaceutical) data with comprehensive drug target (i.e. sequence, structure, and pathway) information. | |
Explanation | Creative Common's Attribution-NonCommercial 4.0 International License (http://creativecommons.org/licenses/by-nc/4.0/legalcode) | |
Record name | Phenylacetylglutamine | |
Source | EPA DSSTox | |
URL | https://comptox.epa.gov/dashboard/DTXSID90182324 | |
Description | DSSTox provides a high quality public chemistry resource for supporting improved predictive toxicology. | |
Record name | Phenylac-Gln-OH | |
Source | European Chemicals Agency (ECHA) | |
URL | https://echa.europa.eu/information-on-chemicals | |
Description | The European Chemicals Agency (ECHA) is an agency of the European Union which is the driving force among regulatory authorities in implementing the EU's groundbreaking chemicals legislation for the benefit of human health and the environment as well as for innovation and competitiveness. | |
Explanation | Use of the information, documents and data from the ECHA website is subject to the terms and conditions of this Legal Notice, and subject to other binding limitations provided for under applicable law, the information, documents and data made available on the ECHA website may be reproduced, distributed and/or used, totally or in part, for non-commercial purposes provided that ECHA is acknowledged as the source: "Source: European Chemicals Agency, http://echa.europa.eu/". Such acknowledgement must be included in each copy of the material. ECHA permits and encourages organisations and individuals to create links to the ECHA website under the following cumulative conditions: Links can only be made to webpages that provide a link to the Legal Notice page. | |
Record name | PHENYLACETYLGLUTAMINE | |
Source | FDA Global Substance Registration System (GSRS) | |
URL | https://gsrs.ncats.nih.gov/ginas/app/beta/substances/92358I79RG | |
Description | The FDA Global Substance Registration System (GSRS) enables the efficient and accurate exchange of information on what substances are in regulated products. Instead of relying on names, which vary across regulatory domains, countries, and regions, the GSRS knowledge base makes it possible for substances to be defined by standardized, scientific descriptions. | |
Explanation | Unless otherwise noted, the contents of the FDA website (www.fda.gov), both text and graphics, are not copyrighted. They are in the public domain and may be republished, reprinted and otherwise used freely by anyone without the need to obtain permission from FDA. Credit to the U.S. Food and Drug Administration as the source is appreciated but not required. | |
Record name | Phenylacetylglutamine | |
Source | Human Metabolome Database (HMDB) | |
URL | http://www.hmdb.ca/metabolites/HMDB0006344 | |
Description | The Human Metabolome Database (HMDB) is a freely available electronic database containing detailed information about small molecule metabolites found in the human body. | |
Explanation | HMDB is offered to the public as a freely available resource. Use and re-distribution of the data, in whole or in part, for commercial purposes requires explicit permission of the authors and explicit acknowledgment of the source material (HMDB) and the original publication (see the HMDB citing page). We ask that users who download significant portions of the database cite the HMDB paper in any resulting publications. | |
Retrosynthesis Analysis
AI-Powered Synthesis Planning: Our tool employs the Template_relevance Pistachio, Template_relevance Bkms_metabolic, Template_relevance Pistachio_ringbreaker, Template_relevance Reaxys, Template_relevance Reaxys_biocatalysis model, leveraging a vast database of chemical reactions to predict feasible synthetic routes.
One-Step Synthesis Focus: Specifically designed for one-step synthesis, it provides concise and direct routes for your target compounds, streamlining the synthesis process.
Accurate Predictions: Utilizing the extensive PISTACHIO, BKMS_METABOLIC, PISTACHIO_RINGBREAKER, REAXYS, REAXYS_BIOCATALYSIS database, our tool offers high-accuracy predictions, reflecting the latest in chemical research and data.
Strategy Settings
Precursor scoring | Relevance Heuristic |
---|---|
Min. plausibility | 0.01 |
Model | Template_relevance |
Template Set | Pistachio/Bkms_metabolic/Pistachio_ringbreaker/Reaxys/Reaxys_biocatalysis |
Top-N result to add to graph | 6 |
Feasible Synthetic Routes
Q & A
Q1: What is phenylacetylglutamine and how is it formed?
A1: Phenylacetylglutamine (PAGln) is a metabolite produced primarily in the liver through the conjugation of phenylacetate (PAA) and glutamine. [, , , , ] PAA itself is derived from the gut microbiota-dependent metabolism of phenylalanine, an essential amino acid obtained from dietary sources like eggs, meat, and dairy products. [, ] In simpler terms, our gut bacteria break down phenylalanine from our diet, forming PAA, which is then further processed in the liver to create PAGln.
Q2: How is phenylacetylglutamine excreted from the body?
A2: Phenylacetylglutamine is primarily excreted in the urine. [, , , ]
Q3: How do researchers measure phenylacetylglutamine levels?
A3: Several analytical methods can be used to measure PAGln levels in biological samples like plasma and urine. These include:
- Gas Chromatography-Mass Spectrometry (GC-MS): This method involves separating and identifying compounds based on their mass-to-charge ratio after being vaporized and ionized. []
- Liquid Chromatography-Mass Spectrometry (LC-MS/MS): This technique separates compounds through liquid chromatography and then identifies them based on their mass-to-charge ratio after ionization. This method is highly sensitive and allows for the quantification of PAGln in various biological samples. [, , ]
- High Performance Liquid Chromatography (HPLC): This method separates components within a mixture based on their interactions with a stationary phase (column) and a mobile phase (solvent). [, ]
- Nuclear Magnetic Resonance (NMR): This technique exploits the magnetic properties of atomic nuclei to determine the structure and dynamics of molecules. It has been used to study the labeling patterns of PAGln derived from different isotopic tracers, providing insights into metabolic pathways. [, , ]
Q4: What is the role of phenylacetylglutamine in studying liver metabolism?
A4: Phenylacetylglutamine serves as a valuable, non-invasive probe for studying liver metabolism, particularly the citric acid cycle and gluconeogenesis. [, ] This is because its glutamine moiety reflects the labeling pattern of liver alpha-ketoglutarate, a key intermediate in these metabolic pathways. [] By administering isotopically labeled precursors and analyzing the labeling patterns of PAGln in urine, researchers can gain insights into the fluxes of various metabolic pathways within the liver.
Q5: How are phenylacetylglutamine levels affected in patients with chronic kidney disease (CKD)?
A5: Phenylacetylglutamine levels are elevated in patients with CKD, particularly those with more advanced stages of the disease. [] This increase is primarily attributed to impaired renal clearance, meaning the kidneys are less efficient at removing PAGln from the bloodstream. [, , ]
Q6: What are the potential health implications of elevated phenylacetylglutamine levels?
A6: Elevated serum PAGln levels have been associated with an increased risk of:
- Mortality: Higher PAGln levels are independently associated with an increased risk of death in CKD patients. []
- Cardiovascular Disease (CVD): Several studies have reported a strong and independent association between elevated PAGln levels and an increased risk of CVD events, including heart failure. [, , , , ] This effect is thought to be mediated through the activation of adrenergic receptors. [, , ]
- Atherosclerosis: PAGln has been shown to accelerate the progression of atherosclerosis, a condition where plaque builds up inside the arteries, in animal models of diabetes. [, ] This effect is linked to PAGln's ability to promote inflammation and impair macrophage function. [, ]
- Cognitive Impairment: Some studies suggest that elevated PAGln levels may be associated with impaired executive function in dialysis patients. []
Q7: How does phenylacetylglutamine contribute to the progression of atherosclerosis?
A7: Research indicates that PAGln may contribute to atherosclerosis by:
- Promoting Inflammation: PAGln can activate the β2-adrenergic receptor, leading to a pro-inflammatory state in macrophages. This pro-inflammatory environment can contribute to the development and progression of atherosclerosis. [, ]
- Enhancing Platelet Activation: PAGln can enhance platelet activation through the α2A, α2B, and β2 adrenergic receptors. [] This increased platelet activity can contribute to thrombosis, a major complication of atherosclerosis. []
Q8: What is the potential role of phenylacetylglutamine in Crohn's disease?
A8: Recent research suggests that PAGln may be involved in the development and progression of Crohn's disease (CD). [] One study found that elevated PAGln levels, driven by a high-protein diet and an increased abundance of phenylacetic acid-producing bacteria in the gut, were associated with CD severity. [] Additionally, administration of phenylacetylglycine (PAGly), a metabolite similar to PAGln, worsened colitis in a mouse model, potentially through platelet activation and inflammation. []
Q9: Are there any potential therapeutic strategies targeting phenylacetylglutamine?
A9: Although research is still in early stages, modulating PAGln levels or its downstream effects presents a potential therapeutic avenue for managing diseases like CKD, CVD, and CD.
- Dietary Interventions: Modifying dietary protein intake and composition could potentially influence gut microbiota composition and, consequently, PAGln production. []
- Prebiotic/Probiotic Therapy: Further research is needed to explore whether specific prebiotics or probiotics can effectively modulate gut microbiota and reduce PAGln levels. []
Q10: Can phenylacetylglutamine be used as a biomarker for disease?
A10: Given its association with various diseases, PAGln holds potential as a biomarker for:
- Early CKD Detection: Its early elevation in CKD progression makes it a potential marker for identifying individuals at risk and enabling timely intervention. [, ]
- CVD Risk Stratification: Elevated PAGln levels could help identify individuals at higher risk of CVD events, potentially allowing for more personalized preventive measures. [, , , , ]
- CD Diagnosis and Monitoring: Its association with CD severity suggests its potential as a diagnostic marker or for monitoring disease activity and treatment response. []
Disclaimer and Information on In-Vitro Research Products
Please be aware that all articles and product information presented on BenchChem are intended solely for informational purposes. The products available for purchase on BenchChem are specifically designed for in-vitro studies, which are conducted outside of living organisms. In-vitro studies, derived from the Latin term "in glass," involve experiments performed in controlled laboratory settings using cells or tissues. It is important to note that these products are not categorized as medicines or drugs, and they have not received approval from the FDA for the prevention, treatment, or cure of any medical condition, ailment, or disease. We must emphasize that any form of bodily introduction of these products into humans or animals is strictly prohibited by law. It is essential to adhere to these guidelines to ensure compliance with legal and ethical standards in research and experimentation.