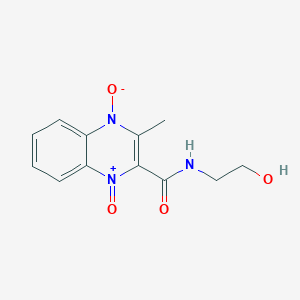
Olaquindox
Overview
Description
Olaquindox is a quinoxaline 1,4-dioxide derivative with antimicrobial properties. It has been primarily used as a growth promoter in pigs and has shown effectiveness in controlling pig dysentery and bacterial enteritis . its use has been banned in the European Union due to concerns about its genotoxicity and potential health risks .
Mechanism of Action
Target of Action
Olaquindox, a quinoxaline 1,4-dioxide derivative, primarily targets the DNA in cells . It interacts with the growth arrest and DNA damage 45 alpha (GADD45a) in human hepatoma G2 cells . It also targets Sertoli cells, which play a crucial role in maintaining mammalian spermatogenesis .
Mode of Action
This compound induces reactive oxygen species (ROS)-mediated DNA damage and S-phase arrest . This process involves the increase of GADD45a, cyclin A, Cdk 2, p21, and p53 protein expression, decrease of cyclin D1, and the activation of phosphorylation-c-Jun N-terminal kinases (p-JNK), phosphorylation-p38 (p-p38), and phosphorylation-extracellular signal-regulated kinases (p-ERK) . When gadd45a is knocked down, this compound significantly decreases cell viability, exacerbates dna damage, and increases s-phase arrest .
Biochemical Pathways
This compound affects the JNK/p38 pathways . GADD45a plays a protective role in this compound treatment, and these pathways may partly contribute to GADD45a regulated this compound-induced DNA damage and S-phase arrest .
Pharmacokinetics
After oral administration of this compound at the rate of 50 mg kg −1 feed for 14 consecutive days in pigs and broilers, the depletion profiles of this compound and its six major metabolites were studied . The elimination half-lives of deoxythis compound residue in broilers’ liver and kidney tissues ( t1/2 >4 days) were much longer than those in pigs .
Result of Action
This compound exposure results in DNA damage, S-phase arrest, and apoptosis in cells . It also disrupts tight junction integrity and cytoskeleton architecture in mouse Sertoli cells .
Action Environment
Environmental factors can influence the action of this compound. For instance, in aquaculture, nearly all of the animals excrete into the water environment directly. As a result, 70%–80% of this compound end up in the environment . This could potentially expose humans and other organisms to the risk of biological hazards due to its genotoxicity and cytotoxicity .
Biochemical Analysis
Biochemical Properties
Olaquindox plays a significant role in biochemical reactions by interacting with various enzymes, proteins, and other biomolecules. It is known to inhibit bacterial DNA synthesis by targeting the bacterial enzyme DNA gyrase. This interaction disrupts the supercoiling of bacterial DNA, leading to the inhibition of bacterial replication and transcription . Additionally, this compound has been shown to interact with other bacterial proteins, contributing to its broad-spectrum antibacterial activity .
Cellular Effects
This compound exerts various effects on different types of cells and cellular processes. In bacterial cells, it inhibits cell division and growth by interfering with DNA synthesis. In mammalian cells, this compound has been reported to induce oxidative stress, leading to the generation of reactive oxygen species (ROS). This oxidative stress can result in DNA damage, lipid peroxidation, and protein oxidation, ultimately affecting cell signaling pathways, gene expression, and cellular metabolism .
Molecular Mechanism
The molecular mechanism of this compound involves its binding interactions with bacterial DNA gyrase, leading to the inhibition of DNA supercoiling. This inhibition prevents the proper replication and transcription of bacterial DNA, resulting in bacterial cell death. Additionally, this compound can induce oxidative stress in mammalian cells by generating reactive oxygen species, which can cause damage to cellular components and alter gene expression .
Temporal Effects in Laboratory Settings
In laboratory settings, the effects of this compound can change over time. This compound is relatively stable under standard laboratory conditions, but it can degrade over time, especially when exposed to light and heat. Long-term exposure to this compound has been shown to cause persistent oxidative stress and DNA damage in mammalian cells, leading to potential long-term effects on cellular function .
Dosage Effects in Animal Models
The effects of this compound vary with different dosages in animal models. At low doses, this compound promotes growth and improves feed efficiency in animals. At high doses, it can cause toxic effects, including liver and kidney damage, as well as oxidative stress and DNA damage. Threshold effects have been observed, where the beneficial effects of this compound are seen at lower doses, while toxic effects become prominent at higher doses .
Metabolic Pathways
This compound is metabolized in the liver, where it undergoes biotransformation to form various metabolites. One of the major metabolites is methyl-3-quinoxaline-2-carboxylic acid, which is used as a biomarker for this compound exposure. The metabolism of this compound involves enzymes such as cytochrome P450, which catalyze the oxidation and reduction reactions. These metabolic pathways can affect the levels of metabolites and influence the overall metabolic flux .
Transport and Distribution
This compound is transported and distributed within cells and tissues through various transporters and binding proteins. It can accumulate in specific tissues, such as the liver and kidneys, where it exerts its effects. The transport and distribution of this compound can influence its localization and accumulation, affecting its overall activity and function .
Subcellular Localization
The subcellular localization of this compound is primarily in the cytoplasm, where it interacts with bacterial DNA gyrase and other proteins. In mammalian cells, this compound can localize to the mitochondria, where it induces oxidative stress and affects mitochondrial function. The targeting signals and post-translational modifications of this compound can direct it to specific compartments or organelles, influencing its activity and function .
Preparation Methods
Olaquindox can be synthesized through various methods. One common synthetic route involves the reaction of 3-methylquinoxaline-2-carboxylic acid with hydroxylamine to form the corresponding oxime, which is then oxidized to produce this compound . Industrial production methods often involve large-scale synthesis using similar reaction conditions, with careful control of temperature and pH to ensure high yield and purity .
Chemical Reactions Analysis
Olaquindox undergoes several types of chemical reactions, including oxidation, reduction, and substitution. It is known to form reactive oxaziridine derivatives upon exposure to light, which can further react with proteins to form photoallergens . Common reagents used in these reactions include oxidizing agents like hydrogen peroxide and reducing agents such as sodium borohydride . Major products formed from these reactions include various metabolites that are extensively metabolized in animals .
Scientific Research Applications
Olaquindox has been widely studied for its antimicrobial properties and its potential genotoxicity. It has been used in research to understand its effects on DNA damage and cell cycle arrest in human hepatoma cells . Additionally, it has been investigated for its role in inducing apoptosis and autophagy in human embryonic kidney cells .
Comparison with Similar Compounds
Olaquindox is structurally related to other quinoxaline 1,4-dioxide derivatives such as carbadox, mequindox, and quinocetone . These compounds share similar antimicrobial properties and mechanisms of action but differ in their potency and toxicity . For example, carbadox is used at lower concentrations compared to this compound but has similar toxic effects . This compound is unique in its ability to form reactive oxaziridine derivatives, which contribute to its photoallergic properties .
Biological Activity
Olaquindox is a synthetic compound primarily used as a veterinary feed additive, particularly in pig farming. Its biological activity has raised concerns due to potential toxicity and adverse effects on animal health, as well as implications for human health through the food chain. This article reviews the biological activity of this compound, focusing on its mechanisms of action, metabolic pathways, and associated toxicological effects.
This compound exhibits its biological activity through several mechanisms, primarily involving oxidative stress and apoptosis in cells.
Oxidative Stress Induction
Research indicates that this compound significantly enhances the production of reactive oxygen species (ROS) in various cell types, including human embryonic kidney (HEK293) cells. A study demonstrated that treatment with this compound led to a dose-dependent increase in ROS levels, malondialdehyde (MDA), and a decrease in antioxidant enzyme activities such as catalase (CAT) and glutathione (GSH) . These changes are indicative of oxidative stress, which can lead to cellular damage and apoptosis.
Table 1: Effects of this compound on Oxidative Stress Markers
Treatment Concentration (μg/mL) | ROS Production (%) | MDA Levels (%) | CAT Activity (%) | GSH Levels (%) |
---|---|---|---|---|
Control | 0 | 100 | 100 | 100 |
400 | +50 | +161.2 | -24.4 | -31.6 |
800 | +100 | +189.48 | -36.2 | -43.8 |
Apoptosis
This compound has been shown to induce apoptosis through the activation of caspases, particularly caspase-3/7, in a dose-dependent manner. This apoptotic effect is linked to the upregulation of autophagy markers such as Beclin 1 and LC3II/LC3I and the downregulation of p70S6K phosphorylation . The interplay between ROS generation and apoptosis suggests that oxidative stress is a critical mediator of this compound-induced cell death.
Metabolism and Tissue Distribution
This compound undergoes extensive biotransformation in vivo, resulting in several metabolites. A study conducted on pigs revealed that this compound is metabolized into six major metabolites through reduction, hydrolysis, and oxidation processes . The tissue distribution of these metabolites was assessed using high-performance liquid chromatography (HPLC), showing higher concentrations of deoxythis compound in muscle, liver, and kidney tissues compared to other metabolites.
Table 2: Tissue Concentrations of this compound Metabolites in Pigs
Tissue | Deoxythis compound (ppm) | MQCA (ppm) |
---|---|---|
Muscle | Higher | Lower |
Liver | Higher | Lower |
Kidney | Higher | Lower |
Hepatotoxicity
This compound has been associated with liver damage in various animal models. In a mouse model study, administration of this compound resulted in significant liver injury characterized by elevated oxidative stress markers and activation of p53 pathways . This suggests a strong link between this compound exposure and hepatotoxicity.
Other Toxic Effects
In addition to hepatotoxicity, this compound has been reported to cause nephrotoxicity and potential mutagenicity. The compound's ability to induce oxidative stress contributes to its overall toxic profile, leading to adverse effects on various organ systems .
Case Study 1: Pigs Treated with this compound
A field study involving pigs treated with this compound at recommended dietary levels reported cases of intoxication characterized by retarded growth and dehydration . Histological examinations revealed changes consistent with adrenal impairment and electrolyte imbalances.
Case Study 2: Long-term Exposure Effects
Long-term studies indicated that pigs receiving high doses of this compound exhibited significant weight loss and organ weight changes, particularly in the spleen and adrenal glands . These findings highlight the potential risks associated with prolonged exposure to this compound.
Properties
IUPAC Name |
N-(2-hydroxyethyl)-3-methyl-4-oxido-1-oxoquinoxalin-1-ium-2-carboxamide | |
---|---|---|
Source | PubChem | |
URL | https://pubchem.ncbi.nlm.nih.gov | |
Description | Data deposited in or computed by PubChem | |
InChI |
InChI=1S/C12H13N3O4/c1-8-11(12(17)13-6-7-16)15(19)10-5-3-2-4-9(10)14(8)18/h2-5,16H,6-7H2,1H3,(H,13,17) | |
Source | PubChem | |
URL | https://pubchem.ncbi.nlm.nih.gov | |
Description | Data deposited in or computed by PubChem | |
InChI Key |
TURHTASYUMWZCC-UHFFFAOYSA-N | |
Source | PubChem | |
URL | https://pubchem.ncbi.nlm.nih.gov | |
Description | Data deposited in or computed by PubChem | |
Canonical SMILES |
CC1=C([N+](=O)C2=CC=CC=C2N1[O-])C(=O)NCCO | |
Source | PubChem | |
URL | https://pubchem.ncbi.nlm.nih.gov | |
Description | Data deposited in or computed by PubChem | |
Molecular Formula |
C12H13N3O4 | |
Source | PubChem | |
URL | https://pubchem.ncbi.nlm.nih.gov | |
Description | Data deposited in or computed by PubChem | |
DSSTOX Substance ID |
DTXSID3040726 | |
Record name | Olaquindox | |
Source | EPA DSSTox | |
URL | https://comptox.epa.gov/dashboard/DTXSID3040726 | |
Description | DSSTox provides a high quality public chemistry resource for supporting improved predictive toxicology. | |
Molecular Weight |
263.25 g/mol | |
Source | PubChem | |
URL | https://pubchem.ncbi.nlm.nih.gov | |
Description | Data deposited in or computed by PubChem | |
Physical Description |
Pale yellow solid; [Merck Index] May cause irritation; Inhalation may cause sensitization, asthma symptoms, or breathing difficulties; Can cause photosensitivity; Harmful by ingestion; [Aldrich MSDS] | |
Record name | Olaquindox | |
Source | Haz-Map, Information on Hazardous Chemicals and Occupational Diseases | |
URL | https://haz-map.com/Agents/1241 | |
Description | Haz-Map® is an occupational health database designed for health and safety professionals and for consumers seeking information about the adverse effects of workplace exposures to chemical and biological agents. | |
Explanation | Copyright (c) 2022 Haz-Map(R). All rights reserved. Unless otherwise indicated, all materials from Haz-Map are copyrighted by Haz-Map(R). No part of these materials, either text or image may be used for any purpose other than for personal use. Therefore, reproduction, modification, storage in a retrieval system or retransmission, in any form or by any means, electronic, mechanical or otherwise, for reasons other than personal use, is strictly prohibited without prior written permission. | |
Solubility |
Slightly soluble in water, Insoluble in most organic solvents. | |
Record name | OLAQUINDOX | |
Source | Hazardous Substances Data Bank (HSDB) | |
URL | https://pubchem.ncbi.nlm.nih.gov/source/hsdb/7025 | |
Description | The Hazardous Substances Data Bank (HSDB) is a toxicology database that focuses on the toxicology of potentially hazardous chemicals. It provides information on human exposure, industrial hygiene, emergency handling procedures, environmental fate, regulatory requirements, nanomaterials, and related areas. The information in HSDB has been assessed by a Scientific Review Panel. | |
Color/Form |
Pale yellow crystals | |
CAS No. |
23696-28-8 | |
Record name | Olaquindox | |
Source | CAS Common Chemistry | |
URL | https://commonchemistry.cas.org/detail?cas_rn=23696-28-8 | |
Description | CAS Common Chemistry is an open community resource for accessing chemical information. Nearly 500,000 chemical substances from CAS REGISTRY cover areas of community interest, including common and frequently regulated chemicals, and those relevant to high school and undergraduate chemistry classes. This chemical information, curated by our expert scientists, is provided in alignment with our mission as a division of the American Chemical Society. | |
Explanation | The data from CAS Common Chemistry is provided under a CC-BY-NC 4.0 license, unless otherwise stated. | |
Record name | Olaquindox | |
Source | EPA DSSTox | |
URL | https://comptox.epa.gov/dashboard/DTXSID3040726 | |
Description | DSSTox provides a high quality public chemistry resource for supporting improved predictive toxicology. | |
Record name | Olaquindox | |
Source | European Chemicals Agency (ECHA) | |
URL | https://echa.europa.eu/substance-information/-/substanceinfo/100.041.651 | |
Description | The European Chemicals Agency (ECHA) is an agency of the European Union which is the driving force among regulatory authorities in implementing the EU's groundbreaking chemicals legislation for the benefit of human health and the environment as well as for innovation and competitiveness. | |
Explanation | Use of the information, documents and data from the ECHA website is subject to the terms and conditions of this Legal Notice, and subject to other binding limitations provided for under applicable law, the information, documents and data made available on the ECHA website may be reproduced, distributed and/or used, totally or in part, for non-commercial purposes provided that ECHA is acknowledged as the source: "Source: European Chemicals Agency, http://echa.europa.eu/". Such acknowledgement must be included in each copy of the material. ECHA permits and encourages organisations and individuals to create links to the ECHA website under the following cumulative conditions: Links can only be made to webpages that provide a link to the Legal Notice page. | |
Record name | OLAQUINDOX | |
Source | FDA Global Substance Registration System (GSRS) | |
URL | https://gsrs.ncats.nih.gov/ginas/app/beta/substances/G3LAW9U88T | |
Description | The FDA Global Substance Registration System (GSRS) enables the efficient and accurate exchange of information on what substances are in regulated products. Instead of relying on names, which vary across regulatory domains, countries, and regions, the GSRS knowledge base makes it possible for substances to be defined by standardized, scientific descriptions. | |
Explanation | Unless otherwise noted, the contents of the FDA website (www.fda.gov), both text and graphics, are not copyrighted. They are in the public domain and may be republished, reprinted and otherwise used freely by anyone without the need to obtain permission from FDA. Credit to the U.S. Food and Drug Administration as the source is appreciated but not required. | |
Record name | OLAQUINDOX | |
Source | Hazardous Substances Data Bank (HSDB) | |
URL | https://pubchem.ncbi.nlm.nih.gov/source/hsdb/7025 | |
Description | The Hazardous Substances Data Bank (HSDB) is a toxicology database that focuses on the toxicology of potentially hazardous chemicals. It provides information on human exposure, industrial hygiene, emergency handling procedures, environmental fate, regulatory requirements, nanomaterials, and related areas. The information in HSDB has been assessed by a Scientific Review Panel. | |
Melting Point |
209 °C (decomposes) | |
Record name | OLAQUINDOX | |
Source | Hazardous Substances Data Bank (HSDB) | |
URL | https://pubchem.ncbi.nlm.nih.gov/source/hsdb/7025 | |
Description | The Hazardous Substances Data Bank (HSDB) is a toxicology database that focuses on the toxicology of potentially hazardous chemicals. It provides information on human exposure, industrial hygiene, emergency handling procedures, environmental fate, regulatory requirements, nanomaterials, and related areas. The information in HSDB has been assessed by a Scientific Review Panel. | |
Retrosynthesis Analysis
AI-Powered Synthesis Planning: Our tool employs the Template_relevance Pistachio, Template_relevance Bkms_metabolic, Template_relevance Pistachio_ringbreaker, Template_relevance Reaxys, Template_relevance Reaxys_biocatalysis model, leveraging a vast database of chemical reactions to predict feasible synthetic routes.
One-Step Synthesis Focus: Specifically designed for one-step synthesis, it provides concise and direct routes for your target compounds, streamlining the synthesis process.
Accurate Predictions: Utilizing the extensive PISTACHIO, BKMS_METABOLIC, PISTACHIO_RINGBREAKER, REAXYS, REAXYS_BIOCATALYSIS database, our tool offers high-accuracy predictions, reflecting the latest in chemical research and data.
Strategy Settings
Precursor scoring | Relevance Heuristic |
---|---|
Min. plausibility | 0.01 |
Model | Template_relevance |
Template Set | Pistachio/Bkms_metabolic/Pistachio_ringbreaker/Reaxys/Reaxys_biocatalysis |
Top-N result to add to graph | 6 |
Feasible Synthetic Routes
Disclaimer and Information on In-Vitro Research Products
Please be aware that all articles and product information presented on BenchChem are intended solely for informational purposes. The products available for purchase on BenchChem are specifically designed for in-vitro studies, which are conducted outside of living organisms. In-vitro studies, derived from the Latin term "in glass," involve experiments performed in controlled laboratory settings using cells or tissues. It is important to note that these products are not categorized as medicines or drugs, and they have not received approval from the FDA for the prevention, treatment, or cure of any medical condition, ailment, or disease. We must emphasize that any form of bodily introduction of these products into humans or animals is strictly prohibited by law. It is essential to adhere to these guidelines to ensure compliance with legal and ethical standards in research and experimentation.