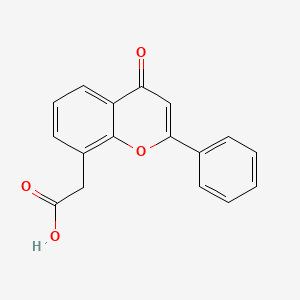
Mitoflaxone
Overview
Description
Mitoflaxone is a small molecule drug with the molecular formula C17H12O4. It is known for its role as a stimulator of interferon genes agonist, which makes it a promising candidate in the field of oncology. The compound was initially developed by Merck Serono SA and has been studied for its potential therapeutic applications in treating neoplasms.
Preparation Methods
The synthesis of Mitoflaxone involves several steps, starting with the preparation of the core structure. The synthetic route typically includes:
Step 1: Formation of the core quinazoline structure through a series of condensation reactions.
Step 2: Introduction of functional groups such as sulfonamide and methyl groups through substitution reactions.
Step 3: Final purification and crystallization to obtain the pure compound.
Industrial production methods may involve optimization of these steps to ensure high yield and purity. Reaction conditions such as temperature, pressure, and the use of catalysts are carefully controlled to achieve the desired product.
Chemical Reactions Analysis
Mitoflaxone undergoes various chemical reactions, including:
Oxidation: The compound can be oxidized to form different derivatives, which may have varying biological activities.
Reduction: Reduction reactions can modify the functional groups, potentially altering the compound’s efficacy.
Substitution: Substitution reactions are used to introduce or replace functional groups, which can enhance the compound’s properties.
Common reagents used in these reactions include oxidizing agents like potassium permanganate, reducing agents such as lithium aluminum hydride, and various nucleophiles for substitution reactions. The major products formed depend on the specific reaction conditions and reagents used.
Scientific Research Applications
Mitoflaxone has been extensively studied for its applications in various fields:
Chemistry: It serves as a model compound for studying the effects of functional group modifications on biological activity.
Biology: The compound is used to investigate cellular pathways and mechanisms, particularly those involving interferon genes.
Medicine: this compound has shown potential in treating neoplasms by activating immune responses through the stimulation of interferon genes.
Industry: The compound’s unique properties make it a candidate for developing new therapeutic agents and diagnostic tools.
Mechanism of Action
Mitoflaxone exerts its effects by acting as a stimulator of interferon genes agonist. This mechanism involves:
Molecular Targets: The primary target is the stimulator of interferon genes protein, which plays a crucial role in the immune response.
Pathways Involved: Activation of the stimulator of interferon genes pathway leads to the production of interferons, which are proteins that help regulate the immune system and combat neoplastic cells.
Comparison with Similar Compounds
Mitoflaxone can be compared with other stimulator of interferon genes agonists such as:
Fluconazole: An antifungal drug that also has immune-modulating properties.
Itaconic Acid: Known for its role in metabolic pathways and immune response.
Quercetin: A flavonoid with antioxidant and anti-inflammatory effects.
This compound is unique due to its specific action on the stimulator of interferon genes pathway, which makes it particularly effective in inducing immune responses against neoplasms.
Biological Activity
Mitoflaxone, a synthetic flavonoid with the molecular formula , is primarily recognized for its potential as an antitumor agent. Developed by Merck Serono SA, it acts as a stimulator of interferon genes (STING) agonist, which plays a crucial role in enhancing immune responses against neoplasms. This compound has garnered attention in both biological and medicinal research due to its unique mechanisms of action and therapeutic applications.
This compound's biological activity is primarily attributed to its ability to activate the STING pathway, leading to the production of interferons. This process involves several key steps:
- Targeting STING Protein : this compound binds to the stimulator of interferon genes protein, initiating a cascade that enhances the immune response.
- Induction of Interferon Production : Activation of this pathway results in increased levels of type I interferons, which are critical for modulating immune responses and combating cancerous cells.
- Impact on Cellular Pathways : The compound influences various cellular pathways associated with apoptosis and cell cycle regulation, making it a candidate for cancer therapy.
Antitumor Effects
Recent studies have demonstrated the antitumor potential of this compound across various cancer models:
- In Vitro Studies : this compound has shown significant cytotoxic effects on several cancer cell lines, including MDA-MB-231 (breast cancer) and A549 (lung cancer) cells. The compound exhibits dose-dependent inhibition of cell viability, indicating its potential as a therapeutic agent.
Cell Line | IC50 (µM) | Effect Observed |
---|---|---|
MDA-MB-231 | 12.5 | Significant cytotoxicity |
A549 | 15.0 | Induction of apoptosis |
- In Vivo Studies : Animal models treated with this compound displayed reduced tumor growth rates compared to control groups. Notably, xenograft models with A549 cells showed substantial tumor suppression when treated with the compound.
Case Studies
- Lung Cancer Model : In a recent study involving A549 xenografts, administration of this compound resulted in a nearly 50% reduction in tumor volume compared to untreated controls. Histological analysis revealed increased apoptosis in tumor tissues.
- Breast Cancer Study : Another investigation focused on MDA-MB-231 cells showed that this compound treatment led to significant cell cycle arrest at the G0/G1 phase, further confirming its role in inhibiting cell proliferation.
Comparative Analysis with Other Compounds
This compound's efficacy can be compared with other known anticancer agents:
Compound | Mechanism | IC50 (µM) | Notes |
---|---|---|---|
This compound | STING agonist | 12.5 | Promotes immune response |
Auranofin | Gold-based mitocan | 25.0 | Repurposed for cancer therapy |
Cisplatin | DNA crosslinker | 10.0 | Traditional chemotherapeutic |
Properties
IUPAC Name |
2-(4-oxo-2-phenylchromen-8-yl)acetic acid | |
---|---|---|
Source | PubChem | |
URL | https://pubchem.ncbi.nlm.nih.gov | |
Description | Data deposited in or computed by PubChem | |
InChI |
InChI=1S/C17H12O4/c18-14-10-15(11-5-2-1-3-6-11)21-17-12(9-16(19)20)7-4-8-13(14)17/h1-8,10H,9H2,(H,19,20) | |
Source | PubChem | |
URL | https://pubchem.ncbi.nlm.nih.gov | |
Description | Data deposited in or computed by PubChem | |
InChI Key |
TZZNWMJZDWYJAZ-UHFFFAOYSA-N | |
Source | PubChem | |
URL | https://pubchem.ncbi.nlm.nih.gov | |
Description | Data deposited in or computed by PubChem | |
Canonical SMILES |
C1=CC=C(C=C1)C2=CC(=O)C3=CC=CC(=C3O2)CC(=O)O | |
Source | PubChem | |
URL | https://pubchem.ncbi.nlm.nih.gov | |
Description | Data deposited in or computed by PubChem | |
Molecular Formula |
C17H12O4 | |
Source | PubChem | |
URL | https://pubchem.ncbi.nlm.nih.gov | |
Description | Data deposited in or computed by PubChem | |
DSSTOX Substance ID |
DTXSID50236534 | |
Record name | Mitoflaxone | |
Source | EPA DSSTox | |
URL | https://comptox.epa.gov/dashboard/DTXSID50236534 | |
Description | DSSTox provides a high quality public chemistry resource for supporting improved predictive toxicology. | |
Molecular Weight |
280.27 g/mol | |
Source | PubChem | |
URL | https://pubchem.ncbi.nlm.nih.gov | |
Description | Data deposited in or computed by PubChem | |
Solubility |
Water < 0.1 (mg/mL), 0.1 N NaOH ~20 (mg/mL), 0.1 N HCl < 0.1 (mg/mL), 0.1 M NaHCO3 < 0.3 (mg/mL), 95% EtOH 1 (mg/mL), MeOH 1 (mg/mL), CHCl3 < 0.1 (mg/mL), DMSO > 100 (mg/mL), TFA > 100 (mg/mL), 10% DMSO < 1 (mg/mL) | |
Record name | FLAVONE ACETIC ACID | |
Source | NCI Investigational Drugs | |
URL | http://dtp.nci.nih.gov/NCI-InvestigationalDrugsCI92/347512%20(1992).txt | |
Description | An investigational drug is one that is under study but does not have permission from the U.S. Food and Drug Administration (FDA) to be legally marketed and sold in the United States. NCI provides the investigational drug to the physicians who are participating in clinical trials or TRC protocols. For more information please visit NCI investigational drug website: https://www.cancer.gov/about-cancer/treatment/drugs/investigational-drug-access-fact-sheet | |
CAS No. |
87626-55-9 | |
Record name | Flavone-8-acetic acid | |
Source | CAS Common Chemistry | |
URL | https://commonchemistry.cas.org/detail?cas_rn=87626-55-9 | |
Description | CAS Common Chemistry is an open community resource for accessing chemical information. Nearly 500,000 chemical substances from CAS REGISTRY cover areas of community interest, including common and frequently regulated chemicals, and those relevant to high school and undergraduate chemistry classes. This chemical information, curated by our expert scientists, is provided in alignment with our mission as a division of the American Chemical Society. | |
Explanation | The data from CAS Common Chemistry is provided under a CC-BY-NC 4.0 license, unless otherwise stated. | |
Record name | Mitoflaxone [INN] | |
Source | ChemIDplus | |
URL | https://pubchem.ncbi.nlm.nih.gov/substance/?source=chemidplus&sourceid=0087626559 | |
Description | ChemIDplus is a free, web search system that provides access to the structure and nomenclature authority files used for the identification of chemical substances cited in National Library of Medicine (NLM) databases, including the TOXNET system. | |
Record name | Flavone acetic acid | |
Source | DTP/NCI | |
URL | https://dtp.cancer.gov/dtpstandard/servlet/dwindex?searchtype=NSC&outputformat=html&searchlist=347512 | |
Description | The NCI Development Therapeutics Program (DTP) provides services and resources to the academic and private-sector research communities worldwide to facilitate the discovery and development of new cancer therapeutic agents. | |
Explanation | Unless otherwise indicated, all text within NCI products is free of copyright and may be reused without our permission. Credit the National Cancer Institute as the source. | |
Record name | Mitoflaxone | |
Source | EPA DSSTox | |
URL | https://comptox.epa.gov/dashboard/DTXSID50236534 | |
Description | DSSTox provides a high quality public chemistry resource for supporting improved predictive toxicology. | |
Record name | MITOFLAXONE | |
Source | FDA Global Substance Registration System (GSRS) | |
URL | https://gsrs.ncats.nih.gov/ginas/app/beta/substances/X230G6E63B | |
Description | The FDA Global Substance Registration System (GSRS) enables the efficient and accurate exchange of information on what substances are in regulated products. Instead of relying on names, which vary across regulatory domains, countries, and regions, the GSRS knowledge base makes it possible for substances to be defined by standardized, scientific descriptions. | |
Explanation | Unless otherwise noted, the contents of the FDA website (www.fda.gov), both text and graphics, are not copyrighted. They are in the public domain and may be republished, reprinted and otherwise used freely by anyone without the need to obtain permission from FDA. Credit to the U.S. Food and Drug Administration as the source is appreciated but not required. | |
Retrosynthesis Analysis
AI-Powered Synthesis Planning: Our tool employs the Template_relevance Pistachio, Template_relevance Bkms_metabolic, Template_relevance Pistachio_ringbreaker, Template_relevance Reaxys, Template_relevance Reaxys_biocatalysis model, leveraging a vast database of chemical reactions to predict feasible synthetic routes.
One-Step Synthesis Focus: Specifically designed for one-step synthesis, it provides concise and direct routes for your target compounds, streamlining the synthesis process.
Accurate Predictions: Utilizing the extensive PISTACHIO, BKMS_METABOLIC, PISTACHIO_RINGBREAKER, REAXYS, REAXYS_BIOCATALYSIS database, our tool offers high-accuracy predictions, reflecting the latest in chemical research and data.
Strategy Settings
Precursor scoring | Relevance Heuristic |
---|---|
Min. plausibility | 0.01 |
Model | Template_relevance |
Template Set | Pistachio/Bkms_metabolic/Pistachio_ringbreaker/Reaxys/Reaxys_biocatalysis |
Top-N result to add to graph | 6 |
Feasible Synthetic Routes
Disclaimer and Information on In-Vitro Research Products
Please be aware that all articles and product information presented on BenchChem are intended solely for informational purposes. The products available for purchase on BenchChem are specifically designed for in-vitro studies, which are conducted outside of living organisms. In-vitro studies, derived from the Latin term "in glass," involve experiments performed in controlled laboratory settings using cells or tissues. It is important to note that these products are not categorized as medicines or drugs, and they have not received approval from the FDA for the prevention, treatment, or cure of any medical condition, ailment, or disease. We must emphasize that any form of bodily introduction of these products into humans or animals is strictly prohibited by law. It is essential to adhere to these guidelines to ensure compliance with legal and ethical standards in research and experimentation.