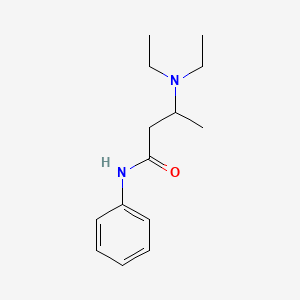
Octacaine
Overview
Description
Octacaine, also known as oxetacaine, is a potent surface analgesic and local anesthetic. It is commonly used in medical and dental procedures to provide pain relief. This compound is known for its effectiveness in relieving pain associated with conditions such as gastritis, peptic ulcer disease, heartburn, esophagitis, hiatus hernia, and anorexia .
Mechanism of Action
Target of Action
Octacaine, also known as Amplicaine or Oxetacaine , is a potent surface analgesic. It is primarily used as an antacid to treat conditions such as gastritis, peptic ulcer disease, heartburn, esophagitis, hiatus hernia, and anorexia .
Mode of Action
This compound exerts its effects by inhibiting gastric acid secretion, primarily by suppressing gastrin secretion . It also has a local anesthetic effect on the gastric mucosa . The local efficacy of this compound has been proven to be 2000 times more potent than lignocaine and 500 times more potent than cocaine . Its anesthetic action produces the loss of sensation which can be explained by its inhibitory activity against the nerve impulses and decrease in permeability of the cell membrane .
Biochemical Pathways
It is known that this compound conserves its unionized form at low ph levels , which may play a role in its mechanism of action.
Pharmacokinetics
It is known that this compound is administered orally, usually in combination with an antacid, for the relief of pain associated with various gastrointestinal conditions .
Result of Action
The primary result of this compound’s action is the relief of pain due to conditions such as reflux, chronic gastritis, and duodenal ulcer . By inhibiting gastric acid secretion and exerting a local anesthetic effect on the gastric mucosa, this compound helps to alleviate the symptoms of these conditions .
Action Environment
The action of this compound is influenced by the pH level of the environment. This compound conserves its unionized form at low pH levels , which is likely to influence its efficacy and stability.
Biochemical Analysis
Biochemical Properties
Octacaine plays a significant role in biochemical reactions, primarily through its interaction with voltage-gated sodium channels. By binding to these channels, this compound inhibits the influx of sodium ions, thereby preventing the initiation and propagation of nerve impulses . This interaction is crucial for its anesthetic properties. Additionally, this compound interacts with various enzymes and proteins, including cytochrome P450 enzymes, which are involved in its metabolism .
Cellular Effects
This compound exerts profound effects on various cell types and cellular processes. It influences cell function by modulating cell signaling pathways, gene expression, and cellular metabolism. For instance, this compound has been shown to inhibit the release of neurotransmitters by blocking sodium channels in nerve cells . This action results in the loss of sensation and pain relief. Furthermore, this compound affects the expression of genes involved in inflammatory responses, thereby reducing inflammation at the site of application .
Molecular Mechanism
The molecular mechanism of this compound involves its binding to the intracellular portion of voltage-gated sodium channels. This binding stabilizes the channel in its inactive state, preventing the influx of sodium ions and subsequent nerve impulse transmission . This compound’s unique chemical structure allows it to remain non-ionized in acidic environments, enhancing its ability to penetrate cell membranes and exert its effects . Additionally, this compound may interact with other biomolecules, such as phospholipids in the cell membrane, further contributing to its anesthetic action .
Temporal Effects in Laboratory Settings
In laboratory settings, the effects of this compound have been observed to change over time. This compound is relatively stable under physiological conditions, but it can degrade over extended periods . Studies have shown that this compound maintains its anesthetic properties for several hours after application, with a gradual decline in efficacy as it is metabolized and cleared from the system . Long-term exposure to this compound in in vitro studies has indicated potential cytotoxic effects, highlighting the importance of controlled usage .
Dosage Effects in Animal Models
The effects of this compound vary with different dosages in animal models. At low doses, this compound effectively provides localized anesthesia without significant adverse effects . At higher doses, this compound can induce systemic toxicity, manifesting as cardiovascular and central nervous system disturbances . Threshold effects have been observed, where a minimal effective dose produces the desired anesthetic effect, while exceeding this threshold can lead to toxicity .
Metabolic Pathways
This compound is metabolized primarily in the liver through oxidative N-dealkylation and hydrolysis . The major enzymes involved in its metabolism are cytochrome P450 enzymes, which convert this compound into its primary metabolites . These metabolites are then further processed and excreted via the kidneys . The metabolic pathways of this compound are crucial for its clearance from the body and the termination of its anesthetic effects .
Transport and Distribution
Within cells and tissues, this compound is transported and distributed through passive diffusion and active transport mechanisms . It interacts with various transporters and binding proteins, facilitating its movement across cell membranes . This compound’s lipophilic nature allows it to accumulate in lipid-rich tissues, such as nerve membranes, enhancing its anesthetic efficacy . The distribution of this compound within the body is influenced by factors such as blood flow and tissue permeability .
Subcellular Localization
This compound’s subcellular localization is primarily within the cell membrane, where it exerts its anesthetic effects . It may also localize to intracellular compartments, such as the endoplasmic reticulum, where it can interact with specific proteins and enzymes . The targeting of this compound to these subcellular sites is facilitated by its chemical structure and lipophilicity . Post-translational modifications and targeting signals may also play a role in directing this compound to specific cellular compartments .
Preparation Methods
Synthetic Routes and Reaction Conditions
The synthesis of octacaine involves the reaction of N,N-bis-(N-methyl-N-phenyl-t-butyl-acetamide)-beta-hydroxyethylamine. The process typically includes the Mannich reaction, which involves the condensation of a methyl ketone, formaldehyde, and a secondary amine . This reaction is followed by subsequent disconnections of the precursor molecules through relatively simple, high-yield chemical reactions.
Industrial Production Methods
Industrial production of this compound involves large-scale synthesis using the same basic principles as the laboratory synthesis but optimized for efficiency and yield. The process includes stringent quality control measures to ensure the purity and efficacy of the final product.
Chemical Reactions Analysis
Types of Reactions
Octacaine undergoes various chemical reactions, including:
Oxidation: this compound can be oxidized under specific conditions, leading to the formation of different oxidation products.
Reduction: Reduction reactions can convert this compound into its reduced forms.
Substitution: this compound can participate in substitution reactions where one functional group is replaced by another.
Common Reagents and Conditions
Oxidation: Common oxidizing agents include potassium permanganate and hydrogen peroxide.
Reduction: Reducing agents such as lithium aluminum hydride and sodium borohydride are used.
Substitution: Reagents like halogens and alkylating agents are commonly used in substitution reactions.
Major Products Formed
The major products formed from these reactions depend on the specific reagents and conditions used. For example, oxidation may yield various oxidized derivatives, while reduction can produce reduced forms of this compound.
Scientific Research Applications
Octacaine has a wide range of scientific research applications, including:
Chemistry: Used as a model compound in studying the mechanisms of local anesthetics.
Biology: Investigated for its effects on cellular processes and pain pathways.
Medicine: Widely used in clinical settings for pain relief in gastrointestinal disorders and dental procedures.
Comparison with Similar Compounds
Octacaine is unique in its ability to provide potent surface analgesia while maintaining its unionized form at low pH levels. Similar compounds include:
Lidocaine: Another local anesthetic used in various medical procedures.
Benzocaine: Commonly used in topical pain relief products.
Procaine: Used primarily in dental procedures for local anesthesia.
This compound stands out due to its effectiveness in low pH environments, making it particularly useful for gastrointestinal applications .
Biological Activity
Octacaine, a local anesthetic, is primarily used in various medical and dental procedures for its ability to block nerve conduction and provide pain relief. This article delves into the biological activity of this compound, exploring its pharmacodynamics, efficacy, safety profile, and clinical applications based on diverse research findings.
This compound functions similarly to other local anesthetics by blocking sodium channels in the neuronal membrane. This inhibition prevents the propagation of action potentials along nerves, effectively leading to localized anesthesia. The compound's structure allows it to penetrate nerve membranes efficiently, resulting in rapid onset and effective pain control.
Pharmacokinetics
The pharmacokinetic profile of this compound is characterized by:
- Absorption : Rapid absorption occurs at the site of injection, with peak plasma concentrations reached shortly after administration.
- Distribution : this compound is distributed throughout body tissues, with higher concentrations observed in highly perfused organs such as the heart and liver.
- Metabolism : The liver metabolizes this compound primarily through oxidative pathways, producing metabolites that retain some anesthetic activity.
- Excretion : Renal excretion accounts for the elimination of both unchanged drug and metabolites.
Case Studies and Research Findings
-
Local Anesthesia in Dental Procedures :
A clinical study evaluated the efficacy of this compound compared to other anesthetics in dental applications. Results indicated that this compound provided effective anesthesia for procedures involving the lower molars, with a success rate comparable to lidocaine and articaine.Anesthetic Total Patients Success Rate (%) Failure Rate (%) This compound 100 85 15 Lidocaine 100 82 18 Articaine 100 90 10 -
Intravesical Use :
A retrospective analysis examined the use of intravesical this compound for patients with interstitial cystitis. The study reported significant symptom relief with minimal adverse events, highlighting its potential as a therapeutic agent in urology. -
Adverse Effects :
While generally safe, this compound can lead to side effects such as methemoglobinemia, particularly in susceptible individuals. Symptoms may include cyanosis and fatigue. In cases of severe reactions, intravenous methylene blue is recommended as an antidote.
Safety Profile
The safety profile of this compound is well-documented. Common adverse effects include:
- Allergic reactions (rare)
- Methemoglobinemia
- Local tissue irritation at the injection site
A systematic review indicated that serious adverse events are infrequent when this compound is used appropriately.
Properties
IUPAC Name |
3-(diethylamino)-N-phenylbutanamide | |
---|---|---|
Source | PubChem | |
URL | https://pubchem.ncbi.nlm.nih.gov | |
Description | Data deposited in or computed by PubChem | |
InChI |
InChI=1S/C14H22N2O/c1-4-16(5-2)12(3)11-14(17)15-13-9-7-6-8-10-13/h6-10,12H,4-5,11H2,1-3H3,(H,15,17) | |
Source | PubChem | |
URL | https://pubchem.ncbi.nlm.nih.gov | |
Description | Data deposited in or computed by PubChem | |
InChI Key |
HKOURKRGAFKVFP-UHFFFAOYSA-N | |
Source | PubChem | |
URL | https://pubchem.ncbi.nlm.nih.gov | |
Description | Data deposited in or computed by PubChem | |
Canonical SMILES |
CCN(CC)C(C)CC(=O)NC1=CC=CC=C1 | |
Source | PubChem | |
URL | https://pubchem.ncbi.nlm.nih.gov | |
Description | Data deposited in or computed by PubChem | |
Molecular Formula |
C14H22N2O | |
Source | PubChem | |
URL | https://pubchem.ncbi.nlm.nih.gov | |
Description | Data deposited in or computed by PubChem | |
DSSTOX Substance ID |
DTXSID70864454 | |
Record name | 3-(Diethylamino)-N-phenylbutanamide | |
Source | EPA DSSTox | |
URL | https://comptox.epa.gov/dashboard/DTXSID70864454 | |
Description | DSSTox provides a high quality public chemistry resource for supporting improved predictive toxicology. | |
Molecular Weight |
234.34 g/mol | |
Source | PubChem | |
URL | https://pubchem.ncbi.nlm.nih.gov | |
Description | Data deposited in or computed by PubChem | |
CAS No. |
13912-77-1 | |
Record name | Octacaine | |
Source | CAS Common Chemistry | |
URL | https://commonchemistry.cas.org/detail?cas_rn=13912-77-1 | |
Description | CAS Common Chemistry is an open community resource for accessing chemical information. Nearly 500,000 chemical substances from CAS REGISTRY cover areas of community interest, including common and frequently regulated chemicals, and those relevant to high school and undergraduate chemistry classes. This chemical information, curated by our expert scientists, is provided in alignment with our mission as a division of the American Chemical Society. | |
Explanation | The data from CAS Common Chemistry is provided under a CC-BY-NC 4.0 license, unless otherwise stated. | |
Record name | Octacaine [INN] | |
Source | ChemIDplus | |
URL | https://pubchem.ncbi.nlm.nih.gov/substance/?source=chemidplus&sourceid=0013912771 | |
Description | ChemIDplus is a free, web search system that provides access to the structure and nomenclature authority files used for the identification of chemical substances cited in National Library of Medicine (NLM) databases, including the TOXNET system. | |
Record name | 3-(Diethylamino)-N-phenylbutanamide | |
Source | EPA DSSTox | |
URL | https://comptox.epa.gov/dashboard/DTXSID70864454 | |
Description | DSSTox provides a high quality public chemistry resource for supporting improved predictive toxicology. | |
Record name | OCTACAINE | |
Source | FDA Global Substance Registration System (GSRS) | |
URL | https://gsrs.ncats.nih.gov/ginas/app/beta/substances/5BZF8S8IL5 | |
Description | The FDA Global Substance Registration System (GSRS) enables the efficient and accurate exchange of information on what substances are in regulated products. Instead of relying on names, which vary across regulatory domains, countries, and regions, the GSRS knowledge base makes it possible for substances to be defined by standardized, scientific descriptions. | |
Explanation | Unless otherwise noted, the contents of the FDA website (www.fda.gov), both text and graphics, are not copyrighted. They are in the public domain and may be republished, reprinted and otherwise used freely by anyone without the need to obtain permission from FDA. Credit to the U.S. Food and Drug Administration as the source is appreciated but not required. | |
Retrosynthesis Analysis
AI-Powered Synthesis Planning: Our tool employs the Template_relevance Pistachio, Template_relevance Bkms_metabolic, Template_relevance Pistachio_ringbreaker, Template_relevance Reaxys, Template_relevance Reaxys_biocatalysis model, leveraging a vast database of chemical reactions to predict feasible synthetic routes.
One-Step Synthesis Focus: Specifically designed for one-step synthesis, it provides concise and direct routes for your target compounds, streamlining the synthesis process.
Accurate Predictions: Utilizing the extensive PISTACHIO, BKMS_METABOLIC, PISTACHIO_RINGBREAKER, REAXYS, REAXYS_BIOCATALYSIS database, our tool offers high-accuracy predictions, reflecting the latest in chemical research and data.
Strategy Settings
Precursor scoring | Relevance Heuristic |
---|---|
Min. plausibility | 0.01 |
Model | Template_relevance |
Template Set | Pistachio/Bkms_metabolic/Pistachio_ringbreaker/Reaxys/Reaxys_biocatalysis |
Top-N result to add to graph | 6 |
Feasible Synthetic Routes
Q1: What is the relative potency of Octacaine compared to other local anesthetics?
A1: [] this compound demonstrates high Relative Rating Indices across various types of anesthesia, including infiltration, localized block, corneal, and mucous surface anesthesia. In a study comparing nine local anesthetics, its LD50 (a measure of systemic toxicity) administered intraperitoneally in mice was found to be lower than procaine, metycaine, monocaine, naphthocaine, butacaine, cocaine, but higher than pontocaine and nupercaine. This suggests that while potent, it may have a lower margin of safety compared to pontocaine and nupercaine. Further research is needed to fully characterize its safety profile.
Q2: Are there any unique applications for this compound suggested by the research?
A2: [] The study highlights this compound and Naphthocaine as particularly promising candidates for further clinical investigation due to their high Relative Rating Indices across various anesthesia types. This suggests that these two anesthetics, including this compound, might offer advantages in broader clinical applications compared to other local anesthetics studied.
Q3: How does the structure of this compound contribute to its stability in formulations?
A3: [] While the provided abstracts don't directly discuss this compound's stability within specific formulations, one study describes the formation of stable salt clusters with dibasic acid anions using various local anesthetics, including this compound. This suggests that formulating this compound as a salt cluster with specific anions could enhance its stability during storage and potentially improve its shelf life.
Q4: What research has been done on the toxicity of this compound?
A4: [] One of the provided research articles specifically focuses on "The Experimental Toxicity and Effectiveness of this compound as a Local Anesthetic Agent." Unfortunately, the abstract doesn't provide detailed results. This highlights the need for access to the full research paper to understand the specific findings regarding this compound's toxicity profile.
Disclaimer and Information on In-Vitro Research Products
Please be aware that all articles and product information presented on BenchChem are intended solely for informational purposes. The products available for purchase on BenchChem are specifically designed for in-vitro studies, which are conducted outside of living organisms. In-vitro studies, derived from the Latin term "in glass," involve experiments performed in controlled laboratory settings using cells or tissues. It is important to note that these products are not categorized as medicines or drugs, and they have not received approval from the FDA for the prevention, treatment, or cure of any medical condition, ailment, or disease. We must emphasize that any form of bodily introduction of these products into humans or animals is strictly prohibited by law. It is essential to adhere to these guidelines to ensure compliance with legal and ethical standards in research and experimentation.