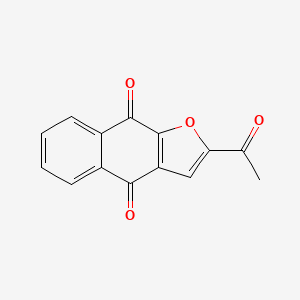
Napabucasin
Overview
Description
Napabucasin (BBI608) is an orally administered small molecule that blocks stem cell activity in cancer cells by targeting the signal transducer and activator of transcription 3 (STAT3) pathway . This pathway is over-activated in many types of cancer and has been shown to be an important pathway in cancer stem cell-mediated propagation of cancer .
Synthesis Analysis
Napabucasin is a novel small molecule inhibitor of STAT3 . It has been identified to eliminate stemness-like tumor cells in some cancers . The synthesis of Napabucasin involves the rational design, synthesis, and biological evaluation of novel potent STAT3 inhibitors based on iso-napabucasin .
Molecular Structure Analysis
Napabucasin belongs to the class of organic compounds known as naphthofurans . These are compounds containing a furan ring fused to a naphthalene moiety. Furan is a 5 membered- ring aromatic ring with four carbon and one oxygen atoms. Naphthalene is a polycyclic aromatic hydrocarbon made up of two fused benzene rings .
Chemical Reactions Analysis
Napabucasin is bioactivated by NQO1, and to a lesser degree by POR, resulting in futile redox cycling and ROS generation . The increased ROS levels result in DNA damage and multiple intracellular changes, one of which is a reduction in STAT3 phosphorylation .
Physical And Chemical Properties Analysis
Napabucasin is a small molecule with a molecular formula of C14H8O4 and a molecular weight of 240.21 .
Scientific Research Applications
Anticancer Effect
Napabucasin, also known as BBI608, is a natural naphthoquinone originally identified as a cancer cell stemness inhibitor . It has shown significant anticancer effects in various types of cancers . The anticancer activities of Napabucasin mainly rely on the inhibition of cancer stemness by targeting signal transducer and activator of transcription 3 (STAT3) and its related gene inhibition .
Inhibition of Cancer Cell Proliferation
Napabucasin has been found to inhibit cancer cell proliferation . This is a crucial aspect of its anticancer activity, as unchecked cell proliferation is a hallmark of cancer.
Induction of Apoptosis
Apoptosis, or programmed cell death, is another mechanism through which Napabucasin exerts its anticancer effects . By inducing apoptosis, Napabucasin can help to eliminate cancer cells and prevent tumor growth.
Cell Cycle Arrest
Napabucasin has been documented in many experiments as a potent cell cycle arrest inducer . By arresting the cell cycle, Napabucasin can prevent cancer cells from dividing and proliferating.
Suppression of Metastasis and Relapse
Napabucasin has been found to suppress metastasis and relapse in cancer patients . This is particularly important, as metastasis and relapse are major challenges in cancer treatment.
Treatment of Glioblastoma
Napabucasin has been identified to eliminate stemness-like tumor cells in some cancers, including glioblastoma . Glioblastoma is a highly aggressive type of brain tumor, and the influence of Napabucasin on glioblastoma cells, especially on glioma stem cells (GSCs), is currently being explored .
Disruption of NF-κB Signaling Pathway
Napabucasin also disrupts the NF-κB signaling pathway via downregulation of RelA (p65) . The NF-κB pathway plays a key role in inflammation and immunity, and its disruption can have significant implications for cancer treatment.
Clinical Trials
Napabucasin is currently being tested in clinical trials for its potential use in cancer treatment . A Phase 3 study of Napabucasin plus standard therapy in colorectal cancer is ongoing .
Mechanism of Action
Target of Action
Napabucasin, also known as BBI608, primarily targets the Signal Transducer and Activator of Transcription 3 (STAT3) . STAT3 is a critical regulator of cancer stem cells (CSCs) in several types of cancer . It is involved in numerous cell signaling pathways implicated in cancer cell survival, proliferation, metastasis, and angiogenesis .
Mode of Action
Napabucasin interacts with its primary target, STAT3, to inhibit its activity . This interaction results in the suppression of cancer cell proliferation, induction of apoptosis and cell cycle arrest, and reduction of metastasis and relapse . Furthermore, Napabucasin has been reported to directly bind to Akt and mTOR proteins, which are upstream regulators of apoptosis and autophagy .
Biochemical Pathways
Napabucasin affects several oncogenic pathways, including the STAT3 pathway . By inhibiting STAT3, Napabucasin suppresses the expression of stemness-associated genes, thereby reducing the stemness of cancer cells . Additionally, Napabucasin disrupts the NF-κB signaling pathway via downregulation of RelA (p65) . It also inhibits other signaling pathways such as Wnt and PI3K/Akt .
Pharmacokinetics
It is known that napabucasin is an orally administered small molecule . In a phase 1 study, Napabucasin was well-tolerated at doses up to 1440 mg/day, and its pharmacokinetic profile was comparable to that reported in previous studies .
Result of Action
Napabucasin’s action results in significant anticancer effects. It suppresses the proliferation, colony formation, and invasion of cancer cells . It also induces cell cycle arrest and apoptosis . More importantly, Napabucasin treatment significantly inhibits the expression of stemness-associated genes, including STAT3, and suppresses the spheroid formation of cancer cells in vitro . In vivo, Napabucasin effectively impairs tumor growth .
Action Environment
It is known that the expression of certain proteins, such as muc1, can influence the sensitivity of cancer cells to napabucasin . Therefore, the tumor microenvironment and the genetic makeup of the cancer cells may play a role in determining the efficacy of Napabucasin.
Safety and Hazards
Future Directions
properties
IUPAC Name |
2-acetylbenzo[f][1]benzofuran-4,9-dione | |
---|---|---|
Source | PubChem | |
URL | https://pubchem.ncbi.nlm.nih.gov | |
Description | Data deposited in or computed by PubChem | |
InChI |
InChI=1S/C14H8O4/c1-7(15)11-6-10-12(16)8-4-2-3-5-9(8)13(17)14(10)18-11/h2-6H,1H3 | |
Source | PubChem | |
URL | https://pubchem.ncbi.nlm.nih.gov | |
Description | Data deposited in or computed by PubChem | |
InChI Key |
DPHUWDIXHNQOSY-UHFFFAOYSA-N | |
Source | PubChem | |
URL | https://pubchem.ncbi.nlm.nih.gov | |
Description | Data deposited in or computed by PubChem | |
Canonical SMILES |
CC(=O)C1=CC2=C(O1)C(=O)C3=CC=CC=C3C2=O | |
Source | PubChem | |
URL | https://pubchem.ncbi.nlm.nih.gov | |
Description | Data deposited in or computed by PubChem | |
Molecular Formula |
C14H8O4 | |
Source | PubChem | |
URL | https://pubchem.ncbi.nlm.nih.gov | |
Description | Data deposited in or computed by PubChem | |
Molecular Weight |
240.21 g/mol | |
Source | PubChem | |
URL | https://pubchem.ncbi.nlm.nih.gov | |
Description | Data deposited in or computed by PubChem | |
CAS RN |
83280-65-3 | |
Record name | Napabucasin [USAN:INN] | |
Source | ChemIDplus | |
URL | https://pubchem.ncbi.nlm.nih.gov/substance/?source=chemidplus&sourceid=0083280653 | |
Description | ChemIDplus is a free, web search system that provides access to the structure and nomenclature authority files used for the identification of chemical substances cited in National Library of Medicine (NLM) databases, including the TOXNET system. | |
Record name | Napabucasin | |
Source | DrugBank | |
URL | https://www.drugbank.ca/drugs/DB12155 | |
Description | The DrugBank database is a unique bioinformatics and cheminformatics resource that combines detailed drug (i.e. chemical, pharmacological and pharmaceutical) data with comprehensive drug target (i.e. sequence, structure, and pathway) information. | |
Explanation | Creative Common's Attribution-NonCommercial 4.0 International License (http://creativecommons.org/licenses/by-nc/4.0/legalcode) | |
Record name | NAPABUCASIN | |
Source | FDA Global Substance Registration System (GSRS) | |
URL | https://gsrs.ncats.nih.gov/ginas/app/beta/substances/Z1HHM49K7O | |
Description | The FDA Global Substance Registration System (GSRS) enables the efficient and accurate exchange of information on what substances are in regulated products. Instead of relying on names, which vary across regulatory domains, countries, and regions, the GSRS knowledge base makes it possible for substances to be defined by standardized, scientific descriptions. | |
Explanation | Unless otherwise noted, the contents of the FDA website (www.fda.gov), both text and graphics, are not copyrighted. They are in the public domain and may be republished, reprinted and otherwise used freely by anyone without the need to obtain permission from FDA. Credit to the U.S. Food and Drug Administration as the source is appreciated but not required. | |
Synthesis routes and methods
Procedure details
Retrosynthesis Analysis
AI-Powered Synthesis Planning: Our tool employs the Template_relevance Pistachio, Template_relevance Bkms_metabolic, Template_relevance Pistachio_ringbreaker, Template_relevance Reaxys, Template_relevance Reaxys_biocatalysis model, leveraging a vast database of chemical reactions to predict feasible synthetic routes.
One-Step Synthesis Focus: Specifically designed for one-step synthesis, it provides concise and direct routes for your target compounds, streamlining the synthesis process.
Accurate Predictions: Utilizing the extensive PISTACHIO, BKMS_METABOLIC, PISTACHIO_RINGBREAKER, REAXYS, REAXYS_BIOCATALYSIS database, our tool offers high-accuracy predictions, reflecting the latest in chemical research and data.
Strategy Settings
Precursor scoring | Relevance Heuristic |
---|---|
Min. plausibility | 0.01 |
Model | Template_relevance |
Template Set | Pistachio/Bkms_metabolic/Pistachio_ringbreaker/Reaxys/Reaxys_biocatalysis |
Top-N result to add to graph | 6 |
Feasible Synthetic Routes
Disclaimer and Information on In-Vitro Research Products
Please be aware that all articles and product information presented on BenchChem are intended solely for informational purposes. The products available for purchase on BenchChem are specifically designed for in-vitro studies, which are conducted outside of living organisms. In-vitro studies, derived from the Latin term "in glass," involve experiments performed in controlled laboratory settings using cells or tissues. It is important to note that these products are not categorized as medicines or drugs, and they have not received approval from the FDA for the prevention, treatment, or cure of any medical condition, ailment, or disease. We must emphasize that any form of bodily introduction of these products into humans or animals is strictly prohibited by law. It is essential to adhere to these guidelines to ensure compliance with legal and ethical standards in research and experimentation.