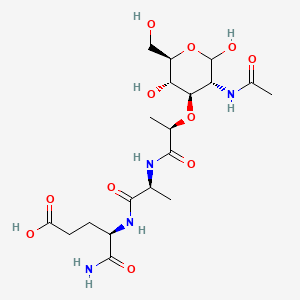
N-Acetylmuramyl-L-alanyl-D-isoglutamine
Overview
Description
Adjuvant peptides are compounds used in vaccines to enhance the body’s immune response to an antigen. These peptides are designed to boost the effectiveness of vaccines by stimulating the immune system, thereby providing better protection against diseases. Adjuvant peptides can be derived from various sources, including synthetic routes and natural extraction, and are crucial in the development of modern vaccines.
Mechanism of Action
Target of Action
Adjuvant peptides primarily target antigen-presenting cells (APCs) . These cells play a crucial role in the immune response by presenting antigens to T cells, thereby initiating an immune response . The peptide adjuvants can also target Toll-like receptors (TLRs) and other pattern recognition receptors (PRRs) on APCs .
Mode of Action
Adjuvant peptides interact with their targets to enhance the immune response. They can be categorized as immunostimulants and delivery systems . As immunostimulants, they act as danger signal molecules that lead to the maturation and activation of APCs by targeting TLRs and other PRRs . This promotes the production of antigen signals and co-stimulatory signals, which in turn enhance the adaptive immune responses . As delivery systems, they facilitate antigen presentation by prolonging the bioavailability of the loaded antigens, as well as targeting antigens to lymph nodes or APCs .
Biochemical Pathways
Adjuvant peptides affect several biochemical pathways to enhance the immune response. They activate innate immune responses, which control the antigen-specific response . They also provide danger signals to activate PRRs on APCs, thereby enhancing antigen presentation on MHC molecules . Furthermore, they promote the generation of antigen presentation signals .
Pharmacokinetics
The pharmacokinetics of adjuvant peptides involve their absorption, distribution, metabolism, and excretion (ADME). It’s important to note that the direct conjugation of a peptide to an adjuvant can enhance the immune response by directly targeting the peptide and adjuvant to the same APC .
Result of Action
The action of adjuvant peptides results in enhanced immune responses. They stimulate the maturation and activation of APCs, promote the production of antigen signals and co-stimulatory signals, and enhance the adaptive immune responses . This can lead to a more robust and effective immune response against the antigen, which is particularly beneficial in the context of vaccines .
Action Environment
The action, efficacy, and stability of adjuvant peptides can be influenced by various environmental factors. For instance, the immune suppressive tumor microenvironment can limit the efficacy of peptide-based cancer vaccines . The use of adjuvants can help to overcome these challenges and improve the efficacy of the vaccines .
Biochemical Analysis
Biochemical Properties
Adjuvant Peptide plays a significant role in biochemical reactions by interacting with various enzymes, proteins, and other biomolecules. It primarily targets Toll-like receptors and other pattern recognition receptors on antigen-presenting cells, leading to the maturation and activation of these cells . This interaction promotes the production of antigen signals and co-stimulatory signals, enhancing adaptive immune responses . Additionally, Adjuvant Peptide can form complexes with lipids and proteins, facilitating their uptake and presentation by immune cells .
Cellular Effects
Adjuvant Peptide influences various types of cells and cellular processes. It enhances the function of antigen-presenting cells, such as dendritic cells and macrophages, by promoting their maturation and activation . This leads to an increased production of cytokines and chemokines, which are crucial for initiating and sustaining immune responses . Adjuvant Peptide also affects cell signaling pathways, gene expression, and cellular metabolism by modulating the activity of transcription factors and other regulatory proteins .
Molecular Mechanism
The molecular mechanism of Adjuvant Peptide involves its binding interactions with biomolecules, enzyme inhibition or activation, and changes in gene expression. Adjuvant Peptide binds to Toll-like receptors and other pattern recognition receptors on the surface of antigen-presenting cells . This binding triggers a cascade of signaling events that lead to the activation of transcription factors, such as NF-κB, which in turn promote the expression of genes involved in immune responses . Additionally, Adjuvant Peptide can inhibit or activate specific enzymes, further modulating cellular functions .
Temporal Effects in Laboratory Settings
In laboratory settings, the effects of Adjuvant Peptide can change over time. The stability and degradation of Adjuvant Peptide are critical factors that influence its long-term effects on cellular function . Studies have shown that Adjuvant Peptide can maintain its stability and activity for extended periods, ensuring sustained immune responses . Prolonged exposure to Adjuvant Peptide may lead to changes in cellular metabolism and function, which need to be carefully monitored .
Dosage Effects in Animal Models
The effects of Adjuvant Peptide vary with different dosages in animal models. At low doses, Adjuvant Peptide effectively enhances immune responses without causing significant adverse effects . At high doses, it may induce toxic or adverse effects, such as inflammation and tissue damage . It is crucial to determine the optimal dosage of Adjuvant Peptide to maximize its benefits while minimizing potential risks .
Metabolic Pathways
Adjuvant Peptide is involved in various metabolic pathways, including those related to immune responses and cellular metabolism . It interacts with enzymes and cofactors that regulate the production of cytokines, chemokines, and other immune mediators . Additionally, Adjuvant Peptide can influence metabolic flux and metabolite levels, further modulating cellular functions .
Transport and Distribution
Adjuvant Peptide is transported and distributed within cells and tissues through interactions with transporters and binding proteins . It can be taken up by antigen-presenting cells and transported to lymph nodes, where it enhances antigen presentation and immune responses . The localization and accumulation of Adjuvant Peptide within specific tissues and cells are crucial for its effectiveness as an adjuvant .
Subcellular Localization
The subcellular localization of Adjuvant Peptide is essential for its activity and function. It can be directed to specific compartments or organelles through targeting signals and post-translational modifications . For example, Adjuvant Peptide may localize to endosomes or lysosomes within antigen-presenting cells, where it interacts with receptors and other biomolecules to enhance immune responses .
Preparation Methods
Synthetic Routes and Reaction Conditions: Adjuvant peptides can be synthesized using solid-phase peptide synthesis (SPPS), a method that allows for the sequential addition of amino acids to a growing peptide chain. The process involves the following steps:
Attachment of the first amino acid: to a solid resin.
Deprotection: of the amino acid’s reactive group.
Coupling: of the next amino acid using coupling reagents like HBTU or DIC.
Repetition: of deprotection and coupling cycles until the desired peptide sequence is obtained.
Cleavage: of the peptide from the resin and purification using techniques like HPLC.
Industrial Production Methods: Industrial production of adjuvant peptides often involves large-scale SPPS or recombinant DNA technology. In recombinant DNA technology, the gene encoding the peptide is inserted into a suitable expression system, such as bacteria or yeast, which then produces the peptide. The peptide is subsequently purified using chromatography techniques.
Chemical Reactions Analysis
Types of Reactions: Adjuvant peptides can undergo various chemical reactions, including:
Oxidation: This reaction can modify the peptide’s structure and function.
Reduction: Often used to break disulfide bonds within the peptide.
Substitution: Involves replacing one amino acid with another to alter the peptide’s properties.
Common Reagents and Conditions:
Oxidizing agents: Hydrogen peroxide, performic acid.
Reducing agents: Dithiothreitol (DTT), beta-mercaptoethanol.
Substitution reagents: Amino acid derivatives, coupling reagents like HBTU.
Major Products: The major products formed from these reactions depend on the specific modifications made to the peptide. For example, oxidation can lead to the formation of sulfoxides or sulfone derivatives, while reduction can yield reduced peptides with free thiol groups.
Scientific Research Applications
Adjuvant peptides have a wide range of applications in scientific research, including:
Chemistry: Used as model compounds to study peptide chemistry and interactions.
Biology: Employed in studies of protein-protein interactions and cellular signaling pathways.
Medicine: Integral components of vaccines, enhancing immune responses to various pathogens.
Industry: Used in the development of diagnostic assays and therapeutic agents.
Comparison with Similar Compounds
Adjuvant peptides can be compared with other adjuvants like aluminum salts, oil-in-water emulsions, and liposomes. While aluminum salts are widely used due to their ability to induce strong antibody responses, adjuvant peptides offer the advantage of being highly specific and capable of inducing both humoral and cellular immune responses. Similar compounds include:
- Aluminum hydroxide
- MF59 (an oil-in-water emulsion)
- Liposome-based adjuvants
Adjuvant peptides stand out due to their ability to be tailored for specific immune responses, making them versatile tools in vaccine development.
Properties
CAS No. |
53678-77-6 |
---|---|
Molecular Formula |
C19H32N4O11 |
Molecular Weight |
492.5 g/mol |
IUPAC Name |
(4R)-4-[[(2S)-2-[[(2R)-2-[(2R,3R,4R,5R)-2-acetamido-4,5,6-trihydroxy-1-oxohexan-3-yl]oxypropanoyl]amino]propanoyl]amino]-5-amino-5-oxopentanoic acid |
InChI |
InChI=1S/C19H32N4O11/c1-8(18(32)23-11(17(20)31)4-5-14(28)29)21-19(33)9(2)34-16(15(30)13(27)7-25)12(6-24)22-10(3)26/h6,8-9,11-13,15-16,25,27,30H,4-5,7H2,1-3H3,(H2,20,31)(H,21,33)(H,22,26)(H,23,32)(H,28,29)/t8-,9+,11+,12-,13+,15+,16+/m0/s1 |
InChI Key |
MEJOVVKHFORGKW-HOMBLFBHSA-N |
Isomeric SMILES |
C[C@@H](C(=O)N[C@H](CCC(=O)O)C(=O)N)NC(=O)[C@@H](C)O[C@H]([C@H](C=O)NC(=O)C)[C@@H]([C@@H](CO)O)O |
SMILES |
CC(C(=O)NC(CCC(=O)O)C(=O)N)NC(=O)C(C)OC1C(C(OC(C1O)CO)O)NC(=O)C |
Canonical SMILES |
CC(C(=O)NC(CCC(=O)O)C(=O)N)NC(=O)C(C)OC(C(C=O)NC(=O)C)C(C(CO)O)O |
Appearance |
Solid powder |
Purity |
>98% (or refer to the Certificate of Analysis) |
shelf_life |
>2 years if stored properly |
solubility |
Soluble in DMSO |
storage |
Dry, dark and at 0 - 4 C for short term (days to weeks) or -20 C for long term (months to years). |
Synonyms |
Acetylmuramyl Alanyl Isoglutamine Acetylmuramyl-Alanyl-Isoglutamine Alanyl Isoglutamine, Acetylmuramyl Dipeptide, Muramyl Isoglutamine, Acetylmuramyl Alanyl Mur NAc L Ala D isoGln Mur-NAc-L-Ala-D-isoGln Muramyl Dipeptide N Acetyl Muramyl L Alanyl D Glutamic alpha Amide N Acetylmuramyl L Alanyl D Isoglutamine N-Acetyl-Muramyl-L-Alanyl-D-Glutamic-alpha-Amide N-Acetylmuramyl-L-Alanyl-D-Isoglutamine |
Origin of Product |
United States |
Retrosynthesis Analysis
AI-Powered Synthesis Planning: Our tool employs the Template_relevance Pistachio, Template_relevance Bkms_metabolic, Template_relevance Pistachio_ringbreaker, Template_relevance Reaxys, Template_relevance Reaxys_biocatalysis model, leveraging a vast database of chemical reactions to predict feasible synthetic routes.
One-Step Synthesis Focus: Specifically designed for one-step synthesis, it provides concise and direct routes for your target compounds, streamlining the synthesis process.
Accurate Predictions: Utilizing the extensive PISTACHIO, BKMS_METABOLIC, PISTACHIO_RINGBREAKER, REAXYS, REAXYS_BIOCATALYSIS database, our tool offers high-accuracy predictions, reflecting the latest in chemical research and data.
Strategy Settings
Precursor scoring | Relevance Heuristic |
---|---|
Min. plausibility | 0.01 |
Model | Template_relevance |
Template Set | Pistachio/Bkms_metabolic/Pistachio_ringbreaker/Reaxys/Reaxys_biocatalysis |
Top-N result to add to graph | 6 |
Feasible Synthetic Routes
Q1: What are adjuvant peptides?
A1: Adjuvant peptides are short amino acid sequences that, when combined with antigens, enhance the immune response against those antigens. [, , ] They are not inherently immunogenic themselves but work by activating various components of the immune system.
Q2: How do adjuvant peptides enhance immune responses?
A2: Adjuvant peptides work through several mechanisms:
- Increased antigen presentation: They can enhance the uptake and presentation of antigens by antigen-presenting cells (APCs), such as dendritic cells and macrophages. [, , ] This is crucial for activating T cells, key players in the adaptive immune response.
- Stimulation of innate immunity: Many adjuvant peptides activate components of the innate immune system, such as Toll-like receptors (TLRs), leading to the production of pro-inflammatory cytokines and chemokines. [, , ] This creates an inflammatory environment that promotes a stronger adaptive immune response.
- Promoting T cell differentiation: Some adjuvant peptides can influence the differentiation of naive T cells into specific subtypes, such as T helper 1 (Th1) or Th2 cells, skewing the immune response towards a desired outcome. [, ]
Q3: What are some examples of adjuvant peptides and their applications?
A3: Here are some notable examples:
- Muramyl dipeptide (MDP): Derived from bacterial cell walls, MDP is a potent activator of the innate immune system, particularly through TLR2. [, , ] It has been investigated for its potential as a vaccine adjuvant and in cancer immunotherapy.
- FK-13: A core peptide derived from antimicrobial peptides, FK-13 has been shown to possess adjuvant properties and was used to develop a fusion peptide (FK-33) with tumor antigen epitopes. [] This fusion peptide, delivered using nanoparticles, demonstrated antitumor efficacy in mice by enhancing antigen presentation and inducing tumor-specific CD8+ T cell responses.
- C5 peptide: Derived from the CFP-10 protein of Mycobacterium tuberculosis, the C5 peptide is a TLR2 agonist. It has been incorporated into an mRNA vaccine candidate, SelmRp25-C5, against tuberculosis. [] This self-adjuvanted vaccine showed promising results in mice, including reduced bacterial burden and increased antigen-specific T cell responses.
Q4: How are adjuvant peptides delivered in vaccines?
A4: Delivery methods for adjuvant peptides are crucial for their effectiveness and can influence the type and strength of the immune response. Common strategies include:
- Direct mixing with antigens: This simple approach involves mixing the adjuvant peptide with the target antigen before administration. []
- Encapsulation in nanoparticles: Incorporating adjuvant peptides into nanoparticles, such as liposomes or polymeric nanoparticles, can improve their stability, target them to specific cells like APCs, and enhance their uptake and processing. [, , , ]
- Chemical conjugation: Covalently linking adjuvant peptides to antigens can enhance their co-delivery to APCs and improve the overall immune response. [, , , ]
- Self-assembling peptide nanofibers: Some adjuvant peptides can self-assemble into nanofibers, forming a depot for sustained antigen release and enhancing immune cell infiltration. [, ]
Q5: What are the advantages of using adjuvant peptides in vaccines?
A5: Adjuvant peptides offer several potential benefits:
- Enhanced immunogenicity: They can significantly boost immune responses to weak antigens, making vaccines more effective. [, , ]
- Improved safety profiles: Compared to some traditional adjuvants, peptide adjuvants often exhibit reduced toxicity and fewer side effects. [, , ]
- Flexibility and specificity: They can be tailored to target specific immune cells or pathways, allowing for fine-tuning of the desired immune response. [, , ]
Q6: What are the challenges and future directions in adjuvant peptide research?
A6: Despite their potential, challenges remain in developing effective and clinically viable adjuvant peptide-based vaccines:
- Understanding structure-activity relationships: Further research is needed to fully understand how the structure of adjuvant peptides influences their activity, potency, and selectivity. [, ]
- Predicting and mitigating potential toxicity: While generally considered safer than some traditional adjuvants, it's essential to thoroughly evaluate the safety and potential long-term effects of adjuvant peptides. [, ]
Q7: What is the role of computational chemistry in adjuvant peptide research?
A7: Computational chemistry plays a vital role in:
- Epitope prediction: Identifying promising peptide sequences with high binding affinities to MHC molecules for improved antigen presentation. [, ]
- Structure-activity relationship (SAR) studies: Analyzing how modifications to the peptide's structure affect its interactions with immune receptors and its overall adjuvanticity. [, ]
- Designing novel adjuvants: Developing new and improved adjuvant peptides with enhanced potency, specificity, and safety profiles. []
Disclaimer and Information on In-Vitro Research Products
Please be aware that all articles and product information presented on BenchChem are intended solely for informational purposes. The products available for purchase on BenchChem are specifically designed for in-vitro studies, which are conducted outside of living organisms. In-vitro studies, derived from the Latin term "in glass," involve experiments performed in controlled laboratory settings using cells or tissues. It is important to note that these products are not categorized as medicines or drugs, and they have not received approval from the FDA for the prevention, treatment, or cure of any medical condition, ailment, or disease. We must emphasize that any form of bodily introduction of these products into humans or animals is strictly prohibited by law. It is essential to adhere to these guidelines to ensure compliance with legal and ethical standards in research and experimentation.