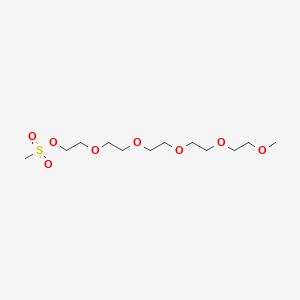
m-PEG6-Ms
Overview
Description
m-PEG6-Ms (methoxy polyethylene glycol hexamer methanesulfonate) is a PROTAC (Proteolysis Targeting Chimera) linker belonging to the polyethylene glycol (PEG) class. It is characterized by a six-unit PEG chain (PEG6) terminated with a methoxy group at one end and a methanesulfonyl (mesyl, Ms) group at the other. The compound (CAS: 130955-39-4, molecular formula: C14H30O9S) is widely utilized in synthesizing PROTAC molecules, which selectively degrade target proteins via the ubiquitin-proteasome system . The mesyl group serves as a reactive handle for nucleophilic substitution reactions, enabling conjugation with E3 ligase ligands or target protein binders in PROTAC design .
Preparation Methods
Synthetic Routes and Reaction Conditions
The synthesis of m-PEG6-Ms typically involves the reaction of methoxy polyethylene glycol with methanesulfonyl chloride in the presence of a base such as triethylamine. The reaction is carried out in an organic solvent like dichloromethane at low temperatures to ensure high yield and purity. The general reaction scheme is as follows:
m-PEG-OH+MsCl→this compound+HCl
Industrial Production Methods
Industrial production of this compound follows similar synthetic routes but on a larger scale. The process involves rigorous purification steps, including recrystallization and chromatography, to achieve high purity levels required for research and pharmaceutical applications .
Chemical Reactions Analysis
Types of Reactions
m-PEG6-Ms primarily undergoes nucleophilic substitution reactions due to the presence of the methanesulfonate group. This group is a good leaving group, making it susceptible to attack by nucleophiles such as thiols, amines, and alcohols.
Common Reagents and Conditions
Nucleophiles: Thiols, amines, alcohols
Solvents: Dichloromethane, tetrahydrofuran
Bases: Triethylamine, pyridine
Conditions: Low temperatures, inert atmosphere
Major Products
The major products formed from these reactions are PEG derivatives with various functional groups, depending on the nucleophile used. For example, reaction with a thiol results in a PEG-thiol compound, while reaction with an amine yields a PEG-amine compound .
Scientific Research Applications
Key Applications
-
Drug Delivery Systems
- m-PEG6-Ms is extensively used in the formulation of drug delivery systems to improve the pharmacokinetics of therapeutic agents. By enhancing solubility and prolonging circulation time in the bloodstream, this compound contributes to improved drug efficacy.
- Case Study : In a study involving PEGylated antibody-drug conjugates (ADCs), formulations using this compound demonstrated significantly higher therapeutic activity against colorectal cancer xenografts compared to non-PEGylated counterparts .
-
Bioconjugation
- The compound serves as a linker for bioconjugation processes, allowing for the attachment of various biomolecules to enhance their functional properties. This is particularly useful in creating targeted therapies that can selectively bind to disease markers.
- Example : this compound has been utilized to link ligands targeting specific proteins with E3 ligase ligands in the development of PROTACs (proteolysis-targeting chimeras). This strategy enables targeted degradation of proteins involved in cancer progression.
-
Nanomedicine
- In nanomedicine, this compound is employed to coat nanoparticles, improving their stability and biocompatibility. This application is crucial for enhancing the delivery of therapeutic agents at the cellular level.
- Data Table :
Application | Description | Benefits |
---|---|---|
Drug Delivery | Use as a linker in ADCs | Improved efficacy and reduced side effects |
Bioconjugation | Attaching biomolecules for targeted therapy | Enhanced specificity and functionality |
Nanoparticle Coating | Stabilization of nanoparticles | Increased solubility and prolonged circulation time |
- Protein Modification
- The compound is also used for modifying proteins through PEGylation, which can reduce immunogenicity and enhance protein stability.
- Research Insight : Studies have shown that PEGylated proteins exhibit improved pharmacokinetic profiles and reduced clearance rates compared to their unmodified forms .
Mechanism of Action
The mechanism of action of m-PEG6-Ms involves the nucleophilic substitution of the methanesulfonate group by various nucleophiles. This reaction results in the formation of PEG derivatives with different functional groups. The PEG backbone provides hydrophilicity and flexibility, while the functional groups impart specific properties to the resulting compounds .
Comparison with Similar Compounds
Structurally analogous PEG-based linkers share the PEG6 backbone but differ in their terminal functional groups, which dictate reactivity, solubility, and application scope. Below is a comparative analysis of m-PEG6-Ms with six closely related compounds (Table 1).
Table 1. Key Properties of this compound and Analogous Compounds
Reactivity and Stability
- This compound : The mesyl group is a superior leaving group for nucleophilic substitutions (e.g., with amines or thiols) due to its small size and high electrophilicity, enabling rapid conjugation under mild conditions. Its stability in aqueous solutions exceeds that of NHS esters, which hydrolyze readily .
- m-PEG6-Tos : The tosyl group, while similarly reactive, is bulkier, leading to slower reaction kinetics. This can be advantageous in controlled syntheses but may limit efficiency in time-sensitive protocols .
- m-PEG6-NHS Ester : Ideal for amine coupling but requires anhydrous conditions to prevent hydrolysis. Unlike this compound, it is unsuitable for thiol-mediated reactions .
Solubility and Biocompatibility
All PEG6 derivatives exhibit high water solubility due to the hydrophilic PEG backbone. However, this compound and m-PEG6-O-CH2COOH demonstrate enhanced solubility in polar organic solvents (e.g., DMSO or DMF), facilitating diverse reaction environments. In contrast, m-PEG6-Br may aggregate in nonpolar solvents due to its hydrophobic bromide terminus .
Application-Specific Advantages
- PROTAC Synthesis : this compound is preferred for its balance of reactivity and stability, enabling efficient linker integration without side reactions. m-PEG6-Azide is favored for copper-catalyzed azide-alkyne cycloaddition (CuAAC) in modular PROTAC assemblies .
Research Findings and Practical Considerations
Recent studies highlight this compound as a versatile linker in PROTACs targeting oncoproteins like BRD4 and EGFR. For example, mesyl-terminated linkers demonstrated 20–30% higher conjugation efficiency compared to tosyl analogues in BRD4 degraders . However, m-PEG6-Azide-based PROTACs showed superior in vivo stability due to the robustness of triazole linkages formed via click chemistry .
Key Limitations :
- This compound may generate methanesulfonic acid as a byproduct, requiring purification steps to avoid interference in biological assays.
- Bromide and tosyl derivatives are less shelf-stable, necessitating storage at –20°C .
Biological Activity
m-PEG6-Ms (methoxy polyethylene glycol 6-mesylate) is a specialized compound utilized primarily in bioconjugation and drug development. This article explores its biological activity, focusing on its role as a linker in the synthesis of PROTACs (Proteolysis Targeting Chimeras), its chemical properties, and its implications in therapeutic applications.
This compound features a polyethylene glycol (PEG) backbone with a mesylate group that facilitates nucleophilic substitution reactions. This property is crucial for linking various ligands to E3 ligases, enabling targeted protein degradation. The general reaction can be illustrated as follows:
Where represents the ligand and signifies the nucleophile. This reaction allows for the selective degradation of proteins involved in disease pathways, particularly in cancer therapy.
Applications in Drug Development
- PROTAC Development : this compound is pivotal in the design of PROTACs, which are engineered to target specific proteins for degradation by the ubiquitin-proteasome system. This approach offers a novel strategy for treating diseases that involve dysregulated protein levels, such as cancer.
- Stability and Reactivity : Research indicates that this compound exhibits stable behavior under physiological conditions, making it suitable for in vivo applications. Studies have focused on optimizing its reactivity to enhance the efficiency of PROTAC synthesis.
Comparative Analysis with Similar Compounds
The following table summarizes the structural features and unique aspects of this compound compared to other PEG derivatives:
Compound Name | Structure/Features | Unique Aspects |
---|---|---|
m-Polyethylene Glycol 6-Bromide | Contains a bromide group | Bromide serves as a good leaving group |
m-Polyethylene Glycol 5-Mesylate | Shorter PEG chain length (5 units) | Different solubility and reactivity profiles |
m-Polyethylene Glycol 8-Mesylate | Longer PEG chain length (8 units) | Potentially improved solubility and pharmacokinetics |
m-Polyethylene Glycol 6-Amino | Contains an amino group | Offers different reactivity profiles for coupling |
This compound stands out due to its mesyl group functionality, which enhances nucleophilic substitution reactions compared to other derivatives.
Case Studies and Research Findings
Several studies have highlighted the efficacy of this compound in various applications:
- Targeted Protein Degradation : In a study focusing on cancer cell lines, PROTACs synthesized using this compound demonstrated significant reductions in target protein levels, leading to decreased cell viability. The targeted approach minimized off-target effects commonly associated with traditional small molecule drugs.
- Antimicrobial Applications : Research has shown that PEGylated antimicrobial peptides exhibit reduced hemolytic activity while maintaining antimicrobial efficacy. The incorporation of this compound into peptide conjugates has demonstrated improved stability against proteolytic degradation, enhancing therapeutic potential against bacterial infections .
Q & A
Basic Research Questions
Q. What experimental design considerations are critical when incorporating m-PEG6-Ms into polymer synthesis protocols?
- Methodological Answer : Use the PICOT framework (Population, Intervention, Comparison, Outcome, Time) to structure variables. For example:
- Population: Target polymer system (e.g., PEGylated nanoparticles).
- Intervention: this compound as a crosslinker or functionalization agent.
- Comparison: Alternative PEGylation agents (e.g., m-PEG4-Ms).
- Outcome: Molecular weight distribution, stability, or bioavailability.
- Time: Reaction duration (e.g., 24–72 hours).
Ensure reproducibility by documenting solvent purity, stoichiometric ratios, and temperature gradients .
Q. How can researchers validate the purity of this compound in synthesized compounds?
- Methodological Answer : Combine HPLC-MS (for molecular weight confirmation) and NMR (for structural integrity). Use triplicate measurements to assess batch-to-batch variability. Reference the Beilstein Journal’s guidelines for reporting characterization data, including retention times, solvent systems, and purity thresholds (>95%) .
Q. What statistical methods are appropriate for analyzing discrepancies in this compound reactivity across studies?
- Methodological Answer : Apply multivariate regression to isolate variables (e.g., solvent polarity, catalyst type). For contradictory data, perform a sensitivity analysis to identify outliers or confounding factors (e.g., trace moisture). Use tools like R or Python’s SciPy for hypothesis testing .
Advanced Research Questions
Q. How can researchers resolve contradictions in reported solubility profiles of this compound across different solvent systems?
- Methodological Answer :
Systematically test solubility in a gradient solvent series (e.g., DCM to DMSO).
Quantify using UV-Vis spectroscopy or dynamic light scattering (DLS).
Cross-reference with Hansen solubility parameters to identify polarity mismatches.
Publish raw data with error margins to enable meta-analysis .
Q. What strategies optimize the stability of this compound in aqueous buffers for long-term biomedical applications?
- Methodological Answer :
- Conduct accelerated stability studies under stress conditions (pH 2–12, 40–60°C).
- Monitor degradation via LC-MS and FTIR to identify hydrolysis or oxidation pathways.
- Use Arrhenius kinetics to extrapolate shelf-life at 25°C .
Q. How should researchers design controls to account for batch-dependent variability in this compound synthesis?
- Methodological Answer :
- Implement a split-plot experimental design :
- Whole-plot factor: Supplier or synthesis batch.
- Sub-plot factors: Reaction temperature, catalyst concentration.
- Use ANOVA to partition variance sources and validate via QbD (Quality by Design) principles .
Q. What ethical and methodological challenges arise when scaling this compound-based drug delivery systems from in vitro to in vivo models?
- Methodological Answer :
- Follow FINER criteria (Feasible, Interesting, Novel, Ethical, Relevant):
- Ethical: Adhere to OECD guidelines for animal testing (e.g., 3Rs principle).
- Feasible: Use pilot studies to determine dosage thresholds.
- Document biocompatibility via histopathology and cytokine profiling .
Q. Data Analysis and Interpretation
Q. How can researchers distinguish between artifacts and genuine spectral signals in this compound characterization?
- Methodological Answer :
- Apply principal component analysis (PCA) to NMR or MS datasets.
- Compare against reference spectra from databases like PubChem or Reaxys.
- Validate with blank experiments (no this compound) to identify solvent/residual peaks .
Q. What frameworks support meta-analysis of conflicting data on this compound cytotoxicity?
- Methodological Answer :
- Use PRISMA guidelines for systematic reviews:
Define inclusion/exclusion criteria (e.g., cell lines, exposure durations).
Perform risk-of-bias assessment via ROBINS-I tool.
Pool data using random-effects models in RevMan or STATA .
Q. Reproducibility and Reporting Standards
Q. What minimal dataset details are required to ensure reproducibility of this compound-related experiments?
- Methodological Answer :
- Report MIAPE (Minimum Information About a Polymer Experiment) standards:
- Synthetic route (e.g., step-by-step protocols).
- Characterization data (e.g., Đ values, retention times).
- Environmental conditions (humidity, light exposure).
- Deposit raw data in repositories like Zenodo or Figshare .
Q. Contradiction Resolution
Q. How should researchers address discrepancies between computational predictions and experimental results for this compound conformation?
- Methodological Answer :
Re-run simulations with explicit solvent models (e.g., TIP3P water).
Validate using SAXS (Small-Angle X-ray Scattering) or cryo-EM.
Publish negative results to inform future force-field parameterization .
Q. Cross-Disciplinary Applications
Q. What interdisciplinary approaches enhance the utility of this compound in hybrid materials research?
Properties
IUPAC Name |
2-[2-[2-[2-(2-methoxyethoxy)ethoxy]ethoxy]ethoxy]ethyl methanesulfonate | |
---|---|---|
Source | PubChem | |
URL | https://pubchem.ncbi.nlm.nih.gov | |
Description | Data deposited in or computed by PubChem | |
InChI |
InChI=1S/C12H26O8S/c1-15-3-4-16-5-6-17-7-8-18-9-10-19-11-12-20-21(2,13)14/h3-12H2,1-2H3 | |
Source | PubChem | |
URL | https://pubchem.ncbi.nlm.nih.gov | |
Description | Data deposited in or computed by PubChem | |
InChI Key |
GIZXGFFADYUCPD-UHFFFAOYSA-N | |
Source | PubChem | |
URL | https://pubchem.ncbi.nlm.nih.gov | |
Description | Data deposited in or computed by PubChem | |
Canonical SMILES |
COCCOCCOCCOCCOCCOS(=O)(=O)C | |
Source | PubChem | |
URL | https://pubchem.ncbi.nlm.nih.gov | |
Description | Data deposited in or computed by PubChem | |
Molecular Formula |
C12H26O8S | |
Source | PubChem | |
URL | https://pubchem.ncbi.nlm.nih.gov | |
Description | Data deposited in or computed by PubChem | |
Molecular Weight |
330.40 g/mol | |
Source | PubChem | |
URL | https://pubchem.ncbi.nlm.nih.gov | |
Description | Data deposited in or computed by PubChem | |
Retrosynthesis Analysis
AI-Powered Synthesis Planning: Our tool employs the Template_relevance Pistachio, Template_relevance Bkms_metabolic, Template_relevance Pistachio_ringbreaker, Template_relevance Reaxys, Template_relevance Reaxys_biocatalysis model, leveraging a vast database of chemical reactions to predict feasible synthetic routes.
One-Step Synthesis Focus: Specifically designed for one-step synthesis, it provides concise and direct routes for your target compounds, streamlining the synthesis process.
Accurate Predictions: Utilizing the extensive PISTACHIO, BKMS_METABOLIC, PISTACHIO_RINGBREAKER, REAXYS, REAXYS_BIOCATALYSIS database, our tool offers high-accuracy predictions, reflecting the latest in chemical research and data.
Strategy Settings
Precursor scoring | Relevance Heuristic |
---|---|
Min. plausibility | 0.01 |
Model | Template_relevance |
Template Set | Pistachio/Bkms_metabolic/Pistachio_ringbreaker/Reaxys/Reaxys_biocatalysis |
Top-N result to add to graph | 6 |
Feasible Synthetic Routes
Disclaimer and Information on In-Vitro Research Products
Please be aware that all articles and product information presented on BenchChem are intended solely for informational purposes. The products available for purchase on BenchChem are specifically designed for in-vitro studies, which are conducted outside of living organisms. In-vitro studies, derived from the Latin term "in glass," involve experiments performed in controlled laboratory settings using cells or tissues. It is important to note that these products are not categorized as medicines or drugs, and they have not received approval from the FDA for the prevention, treatment, or cure of any medical condition, ailment, or disease. We must emphasize that any form of bodily introduction of these products into humans or animals is strictly prohibited by law. It is essential to adhere to these guidelines to ensure compliance with legal and ethical standards in research and experimentation.