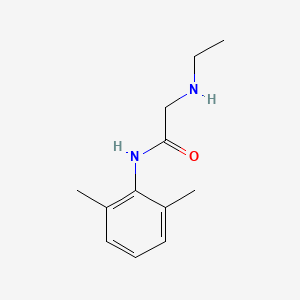
Monoethylglycinexylidide
Overview
Description
Monoethylglycinexylidide (hydrochloride), commonly referred to as MEGX (hydrochloride), is an active metabolite of lidocaine. Lidocaine is a widely used local anesthetic and antiarrhythmic agent. MEGX (hydrochloride) retains pharmacological and toxicological activity similar to lidocaine, although it is less potent. The formation of MEGX (hydrochloride) after lidocaine administration is considered a valid test to evaluate hepatic functionality .
Mechanism of Action
Target of Action
Monoethylglycinexylidide (MEGX), also known as Monoethylglycinexylidine, is primarily a metabolite of the local anesthetic drug Lidocaine . It is formed by the oxidative deethylation of Lidocaine by liver cytochrome P-450 enzymes . Therefore, its primary targets are the same as those of Lidocaine, which are voltage-gated sodium channels in the nervous system.
Mode of Action
MEGX, like Lidocaine, interacts with its targets by binding to the intracellular portion of sodium channels and blocking the influx of sodium ions . This prevents the generation and conduction of nerve impulses, thereby producing a local anesthetic effect.
Biochemical Pathways
The formation of MEGX from Lidocaine involves the biochemical pathway of oxidative deethylation, which is catalyzed by liver cytochrome P-450 enzymes . This process is part of the broader metabolic pathway of drug biotransformation in the liver.
Pharmacokinetics
The pharmacokinetics of MEGX is closely related to that of its parent drug, Lidocaine. Lidocaine has a high extraction ratio in the liver and shows blood-flow-limited clearance . The terminal half-life of Lidocaine increases from a normal 1.4 to 10.2 hours in cardiac failure . Changes in the half-life of MEGX are similar; 2.3 to 7.8 hours . The elimination clearance of Lidocaine and its metabolites, including MEGX, is reduced after a partial hepatectomy .
Result of Action
The primary result of MEGX’s action is the local anesthetic effect produced by the blockage of sodium channels. This leads to a decrease in the excitability of nerve membranes and a loss of sensation in the area where the drug is applied. Additionally, MEGX has been proven to be a highly sensitive indicator of hepatic dysfunction, especially in the field of liver transplantation .
Action Environment
The action of MEGX is influenced by various environmental factors. For instance, the rate of its formation from Lidocaine can be affected by the functional state of the liver, as the cytochrome P-450 enzymes responsible for its formation are located in this organ . Therefore, conditions such as liver disease or cardiac failure, which can affect liver function and blood flow, can potentially influence the action and efficacy of MEGX .
Biochemical Analysis
Biochemical Properties
Monoethylglycinexylidide interacts with liver cytochrome P-450 enzymes during its formation . This interaction involves the oxidative deethylation of lignocaine, a widely used local anesthetic drug .
Cellular Effects
This compound has been found to inhibit GlyT1 function in primary astrocytes and in GlyT1-expressing Xenopus laevis oocytes at clinically relevant concentrations . This inhibition of GlyT1-mediated glycine uptake could potentially influence cell function, including impacts on cell signaling pathways, gene expression, and cellular metabolism .
Molecular Mechanism
This compound exerts its effects at the molecular level through its interactions with cytochrome P-450 enzymes in the liver . It is produced via oxidative N-deethylation of lignocaine . Additionally, it has been found to inhibit GlyT1 function, which could potentially lead to changes in gene expression .
Preparation Methods
Synthetic Routes and Reaction Conditions
MEGX (hydrochloride) is synthesized through the de-ethylation of lidocaine by the cytochrome P450 enzyme system, specifically CYP3A4. This metabolic process involves the removal of an ethyl group from the lidocaine molecule, resulting in the formation of MEGX (hydrochloride) .
Industrial Production Methods
Industrial production of MEGX (hydrochloride) typically involves the use of high-performance liquid chromatography (HPLC) coupled with tandem mass spectrometry (LC-MS-MS) for the determination and purification of the compound. The process includes deproteinization with acetonitrile, followed by solid-phase extraction using strong cation exchange cartridges .
Chemical Reactions Analysis
Types of Reactions
MEGX (hydrochloride) undergoes various chemical reactions, including:
Oxidation: The compound can be further oxidized to form glycine xylidide, an inactive metabolite.
Reduction: Reduction reactions are less common for MEGX (hydrochloride) due to its stable amide structure.
Substitution: The compound can undergo substitution reactions, particularly involving the amide group.
Common Reagents and Conditions
Oxidation: Common oxidizing agents include hydrogen peroxide and potassium permanganate.
Substitution: Reagents such as alkyl halides and acyl chlorides can be used under acidic or basic conditions.
Major Products Formed
Oxidation: Glycine xylidide.
Substitution: Various substituted amides depending on the reagents used.
Scientific Research Applications
MEGX (hydrochloride) has several scientific research applications, including:
Chemistry: Used as a reference standard in analytical chemistry for the determination of lidocaine and its metabolites.
Biology: Employed in studies to evaluate hepatic function and the metabolic capacity of the liver.
Medicine: Utilized in pharmacokinetic studies to assess the metabolism and clearance of lidocaine in patients.
Comparison with Similar Compounds
Similar Compounds
Glycine xylidide: An inactive metabolite of lidocaine formed by further oxidation of MEGX (hydrochloride).
N-ethylglycine: Another metabolite of lidocaine with similar properties.
Uniqueness
MEGX (hydrochloride) is unique due to its intermediate activity between lidocaine and its inactive metabolites. It retains some pharmacological activity, making it useful in evaluating hepatic function and in pharmacokinetic studies .
Properties
IUPAC Name |
N-(2,6-dimethylphenyl)-2-(ethylamino)acetamide | |
---|---|---|
Source | PubChem | |
URL | https://pubchem.ncbi.nlm.nih.gov | |
Description | Data deposited in or computed by PubChem | |
InChI |
InChI=1S/C12H18N2O/c1-4-13-8-11(15)14-12-9(2)6-5-7-10(12)3/h5-7,13H,4,8H2,1-3H3,(H,14,15) | |
Source | PubChem | |
URL | https://pubchem.ncbi.nlm.nih.gov | |
Description | Data deposited in or computed by PubChem | |
InChI Key |
WRMRXPASUROZGT-UHFFFAOYSA-N | |
Source | PubChem | |
URL | https://pubchem.ncbi.nlm.nih.gov | |
Description | Data deposited in or computed by PubChem | |
Canonical SMILES |
CCNCC(=O)NC1=C(C=CC=C1C)C | |
Source | PubChem | |
URL | https://pubchem.ncbi.nlm.nih.gov | |
Description | Data deposited in or computed by PubChem | |
Molecular Formula |
C12H18N2O | |
Source | PubChem | |
URL | https://pubchem.ncbi.nlm.nih.gov | |
Description | Data deposited in or computed by PubChem | |
DSSTOX Substance ID |
DTXSID60228006 | |
Record name | Monoethylglycinexylidide | |
Source | EPA DSSTox | |
URL | https://comptox.epa.gov/dashboard/DTXSID60228006 | |
Description | DSSTox provides a high quality public chemistry resource for supporting improved predictive toxicology. | |
Molecular Weight |
206.28 g/mol | |
Source | PubChem | |
URL | https://pubchem.ncbi.nlm.nih.gov | |
Description | Data deposited in or computed by PubChem | |
Solubility |
25.2 [ug/mL] (The mean of the results at pH 7.4) | |
Record name | SID56320465 | |
Source | Burnham Center for Chemical Genomics | |
URL | https://pubchem.ncbi.nlm.nih.gov/bioassay/1996#section=Data-Table | |
Description | Aqueous solubility in buffer at pH 7.4 | |
CAS No. |
7728-40-7 | |
Record name | Monoethylglycinexylidide | |
Source | CAS Common Chemistry | |
URL | https://commonchemistry.cas.org/detail?cas_rn=7728-40-7 | |
Description | CAS Common Chemistry is an open community resource for accessing chemical information. Nearly 500,000 chemical substances from CAS REGISTRY cover areas of community interest, including common and frequently regulated chemicals, and those relevant to high school and undergraduate chemistry classes. This chemical information, curated by our expert scientists, is provided in alignment with our mission as a division of the American Chemical Society. | |
Explanation | The data from CAS Common Chemistry is provided under a CC-BY-NC 4.0 license, unless otherwise stated. | |
Record name | Monoethylglycinexylidide | |
Source | ChemIDplus | |
URL | https://pubchem.ncbi.nlm.nih.gov/substance/?source=chemidplus&sourceid=0007728407 | |
Description | ChemIDplus is a free, web search system that provides access to the structure and nomenclature authority files used for the identification of chemical substances cited in National Library of Medicine (NLM) databases, including the TOXNET system. | |
Record name | Monoethylglycinexylidide | |
Source | EPA DSSTox | |
URL | https://comptox.epa.gov/dashboard/DTXSID60228006 | |
Description | DSSTox provides a high quality public chemistry resource for supporting improved predictive toxicology. | |
Record name | N-(2,6-dimethylphenyl)-2-(ethylamino)acetamide | |
Source | European Chemicals Agency (ECHA) | |
URL | https://echa.europa.eu/information-on-chemicals | |
Description | The European Chemicals Agency (ECHA) is an agency of the European Union which is the driving force among regulatory authorities in implementing the EU's groundbreaking chemicals legislation for the benefit of human health and the environment as well as for innovation and competitiveness. | |
Explanation | Use of the information, documents and data from the ECHA website is subject to the terms and conditions of this Legal Notice, and subject to other binding limitations provided for under applicable law, the information, documents and data made available on the ECHA website may be reproduced, distributed and/or used, totally or in part, for non-commercial purposes provided that ECHA is acknowledged as the source: "Source: European Chemicals Agency, http://echa.europa.eu/". Such acknowledgement must be included in each copy of the material. ECHA permits and encourages organisations and individuals to create links to the ECHA website under the following cumulative conditions: Links can only be made to webpages that provide a link to the Legal Notice page. | |
Record name | NORLIDOCAINE | |
Source | FDA Global Substance Registration System (GSRS) | |
URL | https://gsrs.ncats.nih.gov/ginas/app/beta/substances/D8Q99HC770 | |
Description | The FDA Global Substance Registration System (GSRS) enables the efficient and accurate exchange of information on what substances are in regulated products. Instead of relying on names, which vary across regulatory domains, countries, and regions, the GSRS knowledge base makes it possible for substances to be defined by standardized, scientific descriptions. | |
Explanation | Unless otherwise noted, the contents of the FDA website (www.fda.gov), both text and graphics, are not copyrighted. They are in the public domain and may be republished, reprinted and otherwise used freely by anyone without the need to obtain permission from FDA. Credit to the U.S. Food and Drug Administration as the source is appreciated but not required. | |
Record name | Monoethylglycinexylidide | |
Source | Human Metabolome Database (HMDB) | |
URL | http://www.hmdb.ca/metabolites/HMDB0060656 | |
Description | The Human Metabolome Database (HMDB) is a freely available electronic database containing detailed information about small molecule metabolites found in the human body. | |
Explanation | HMDB is offered to the public as a freely available resource. Use and re-distribution of the data, in whole or in part, for commercial purposes requires explicit permission of the authors and explicit acknowledgment of the source material (HMDB) and the original publication (see the HMDB citing page). We ask that users who download significant portions of the database cite the HMDB paper in any resulting publications. | |
Synthesis routes and methods
Procedure details
Retrosynthesis Analysis
AI-Powered Synthesis Planning: Our tool employs the Template_relevance Pistachio, Template_relevance Bkms_metabolic, Template_relevance Pistachio_ringbreaker, Template_relevance Reaxys, Template_relevance Reaxys_biocatalysis model, leveraging a vast database of chemical reactions to predict feasible synthetic routes.
One-Step Synthesis Focus: Specifically designed for one-step synthesis, it provides concise and direct routes for your target compounds, streamlining the synthesis process.
Accurate Predictions: Utilizing the extensive PISTACHIO, BKMS_METABOLIC, PISTACHIO_RINGBREAKER, REAXYS, REAXYS_BIOCATALYSIS database, our tool offers high-accuracy predictions, reflecting the latest in chemical research and data.
Strategy Settings
Precursor scoring | Relevance Heuristic |
---|---|
Min. plausibility | 0.01 |
Model | Template_relevance |
Template Set | Pistachio/Bkms_metabolic/Pistachio_ringbreaker/Reaxys/Reaxys_biocatalysis |
Top-N result to add to graph | 6 |
Feasible Synthetic Routes
Q1: How is Monoethylglycinexylidine formation used to assess liver function?
A1: Monoethylglycinexylidine (MEGX) formation serves as a marker for assessing liver function due to its dependence on specific enzymatic activity within the liver. The liver's ability to metabolize certain drugs, including lidocaine, can be impaired during liver disease. The conversion of lidocaine to MEGX is primarily mediated by cytochrome P450 enzymes, specifically CYP3A4, found in the liver. [, ] By administering a known dose of lidocaine and measuring the subsequent levels of MEGX in the bloodstream, clinicians and researchers can gain insights into the liver's metabolic capacity. Reduced MEGX formation suggests impaired liver function and may warrant further investigation to determine the underlying cause of the dysfunction. [, ] This test is particularly valuable in cases where conventional liver function tests may not be sensitive enough to detect subtle changes in liver function or in monitoring the progression of liver disease.
Q2: Does omeprazole, a gastric antisecretory drug, affect the levels of lidocaine and Monoethylglycinexylidine in the body?
A2: Research suggests that omeprazole does not significantly impact the levels of lidocaine or Monoethylglycinexylidine. A study involving healthy volunteers found no significant differences in the areas under the curve (AUC) for both lidocaine and MEGX after pretreatment with omeprazole compared to a placebo. [] This indicates that omeprazole does not appear to alter the overall exposure to lidocaine or its metabolite, MEGX. These findings suggest that omeprazole is unlikely to clinically affect the pharmacokinetics of lidocaine, allowing for the safe co-administration of these drugs without the need for dosage adjustments.
Q3: Can opioids influence lidocaine disposition and potentially lead to toxicity, and what is the role of Monoethylglycinexylidine in this interaction?
A3: Yes, opioids, specifically morphine, meperidine, and fentanyl, have been shown to affect lidocaine disposition and increase its potential for toxicity in mice. [] The study demonstrated that these opioids, particularly morphine, elevated plasma lidocaine levels while reducing the levels of its metabolites, including MEGX and glycinexylidine. [] This effect is believed to be due to reduced hepatic blood flow caused by the opioids, affecting the liver's ability to metabolize lidocaine effectively. The decrease in MEGX levels further supports this hypothesis, as it indicates a reduced rate of lidocaine breakdown. This interaction highlights a potential risk of increased lidocaine toxicity when co-administered with opioids, emphasizing the importance of careful monitoring and potential dosage adjustments to ensure patient safety.
Q4: How is Monoethylglycinexylidine detected and quantified in biological samples?
A4: Monoethylglycinexylidine is typically detected and quantified in biological samples, like plasma or urine, using chromatographic techniques coupled with mass spectrometry. [, ] These methods offer high sensitivity and selectivity, enabling accurate measurement even at low concentrations. The sample preparation usually involves extraction and purification steps to remove interfering compounds. While Enzyme-linked immunosorbent assay (ELISA) can screen for lidocaine and its metabolites, it doesn't specifically identify MEGX. [] Quantitative mass spectrometry proves crucial for accurately determining MEGX concentrations in biological samples. For instance, a study developed and validated a mass spectrometry method to quantify 3-hydroxylidocaine, another lidocaine metabolite, in horse urine. [] Similar methods can be employed for MEGX quantification, providing valuable data for pharmacokinetic studies and assessing liver function.
Q5: What is the significance of Monoethylglycinexylidine levels in the context of chronic liver transplant rejection?
A5: Persistently high cyclosporine levels can occur in patients with chronic liver transplant rejection, potentially due to a down-regulation of the cytochrome P450 enzyme system. [, ] This system is crucial for metabolizing various drugs, including cyclosporine. The MEGX test can confirm this hypothesis by assessing the functionality of the cytochrome P450 system. [] Low MEGX formation after a lidocaine challenge in such patients would support the presence of impaired drug metabolism, explaining the elevated cyclosporine levels. This information can guide clinicians in adjusting immunosuppressive therapy to prevent cyclosporine toxicity and improve long-term graft outcomes.
Disclaimer and Information on In-Vitro Research Products
Please be aware that all articles and product information presented on BenchChem are intended solely for informational purposes. The products available for purchase on BenchChem are specifically designed for in-vitro studies, which are conducted outside of living organisms. In-vitro studies, derived from the Latin term "in glass," involve experiments performed in controlled laboratory settings using cells or tissues. It is important to note that these products are not categorized as medicines or drugs, and they have not received approval from the FDA for the prevention, treatment, or cure of any medical condition, ailment, or disease. We must emphasize that any form of bodily introduction of these products into humans or animals is strictly prohibited by law. It is essential to adhere to these guidelines to ensure compliance with legal and ethical standards in research and experimentation.