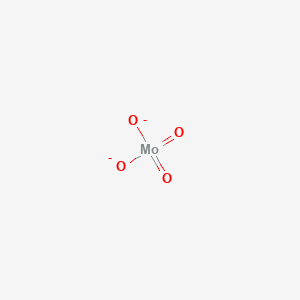
Molybdate
Overview
Description
Molybdate refers to a compound containing an oxyanion with molybdenum in its highest oxidation state of 6: O−−Mo(=O)2−O−. Molybdenum can form a wide range of such oxyanions, which can be discrete structures or polymeric extended structures, although the latter are only found in the solid state . This compound compounds are essential in various chemical, biological, and industrial processes due to their unique properties and versatility.
Synthetic Routes and Reaction Conditions:
Microwave Roasting and Alkali Leaching: This method involves the oxidation roasting of molybdenum concentrate followed by alkali leaching. The optimal conditions include a roasting temperature of 700°C, holding time of 110 minutes, and a power-to-mass ratio of 110 W/g.
Ammonia Leaching: This process involves the ammonia leaching of calcined molybdenum obtained by microwave roasting. The optimal conditions include an ammonia concentration of 25%, a solid-to-liquid ratio of 1/2 g/ml, and a leaching temperature of 75°C.
Industrial Production Methods:
Liquid Alkali Process: This method involves dissolving molybdenum oxide in a sodium hydroxide or sodium carbonate solution to prepare sodium this compound crystals.
Classical Ammonia Leaching: This method includes oxidative roasting of molybdenum concentrate followed by wet treatment, such as extraction, ion exchange, and continuous crystallization.
Types of Reactions:
Substitution: this compound reacts with acids of dithiophosphates to form complex compounds like [MoO2(S2P(OR)2)2] and [MoO3(S2P(OR)2)4].
Common Reagents and Conditions:
Sodium Borohydride: Used for the reduction of this compound to molybdenum (IV) oxide.
Dithiophosphates: React with this compound to form complex compounds.
Major Products:
Molybdenum (IV) Oxide: Formed from the reduction of this compound.
Complex Compounds: Such as [MoO2(S2P(OR)2)2] and [MoO3(S2P(OR)2)4].
Scientific Research Applications
Molybdate compounds have a wide range of applications in scientific research:
Chemistry: Used as catalysts in various chemical reactions, including oxidative dehydrogenation and selective oxidation.
Medicine: Investigated for their potential use in medical imaging and as therapeutic agents.
Industry: Used as corrosion inhibitors in cooling water systems and as components in fertilizers.
Mechanism of Action
Molybdate exerts its effects through its incorporation into molybdenum cofactors, which serve as the active sites of molybdenum-requiring enzymes. These enzymes participate in crucial biological processes, such as nitrate assimilation, phytohormone biosynthesis, and sulfite detoxification . The molecular targets include various molybdoenzymes, and the pathways involved are related to the specific biological functions of these enzymes.
Comparison with Similar Compounds
Chromates: Compounds containing chromium in its highest oxidation state.
Tungstates: Compounds containing tungsten in its highest oxidation state.
Molybdate compounds are unique due to their versatility and wide range of applications in various fields, making them an essential component in scientific research and industrial processes.
Properties
CAS No. |
14259-85-9 |
---|---|
Molecular Formula |
MoO4-2 |
Molecular Weight |
159.95 g/mol |
IUPAC Name |
dioxido(dioxo)molybdenum |
InChI |
InChI=1S/Mo.4O/q;;;2*-1 |
InChI Key |
MEFBJEMVZONFCJ-UHFFFAOYSA-N |
SMILES |
[O-][Mo](=O)(=O)[O-] |
Canonical SMILES |
[O-][Mo](=O)(=O)[O-] |
Appearance |
Solid powder |
Purity |
>98% (or refer to the Certificate of Analysis) |
shelf_life |
>3 years if stored properly |
solubility |
Soluble in DMSO |
storage |
Dry, dark and at 0 - 4 C for short term (days to weeks) or -20 C for long term (months to years). |
Synonyms |
molybdate molybdate (Mo7-O24) molybdate ion molybdate, (99)Mo-labeled MoO4(2-) |
Origin of Product |
United States |
Synthesis routes and methods I
Procedure details
Synthesis routes and methods II
Procedure details
Disclaimer and Information on In-Vitro Research Products
Please be aware that all articles and product information presented on BenchChem are intended solely for informational purposes. The products available for purchase on BenchChem are specifically designed for in-vitro studies, which are conducted outside of living organisms. In-vitro studies, derived from the Latin term "in glass," involve experiments performed in controlled laboratory settings using cells or tissues. It is important to note that these products are not categorized as medicines or drugs, and they have not received approval from the FDA for the prevention, treatment, or cure of any medical condition, ailment, or disease. We must emphasize that any form of bodily introduction of these products into humans or animals is strictly prohibited by law. It is essential to adhere to these guidelines to ensure compliance with legal and ethical standards in research and experimentation.