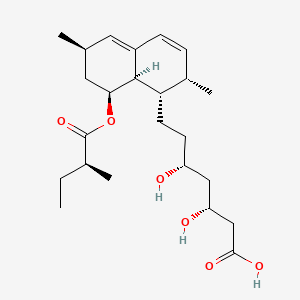
Lovastatin acid
Overview
Description
Mevinolinic acid, also known as the hydroxy-acid form of mevinolin, is a potent competitive inhibitor of 3-hydroxy-3-methylglutaryl-coenzyme A reductase (HMG-CoA reductase). This enzyme plays a crucial role in the biosynthesis of cholesterol. Mevinolinic acid is derived from the fungal metabolite mevinolin, which is produced by Aspergillus terreus .
Mechanism of Action
Lovastatin acid, also known as Mevinolinic acid, is a lipid-lowering drug that belongs to the statin class of medications . It is used to lower the risk of cardiovascular disease and manage abnormal lipid levels by inhibiting the endogenous production of cholesterol in the liver .
Target of Action
Lovastatin’s primary target is the enzyme hydroxymethylglutaryl-coenzyme A (HMG-CoA) Reductase . This enzyme catalyzes the conversion of HMG-CoA to mevalonic acid, a crucial step in cholesterol biosynthesis . By inhibiting this enzyme, lovastatin effectively reduces the production of cholesterol in the body .
Mode of Action
Lovastatin acts by competitively inhibiting HMG-CoA reductase . This means it competes with the enzyme’s natural substrate, HMG-CoA, for binding sites on the enzyme . When lovastatin binds to HMG-CoA reductase, it prevents the enzyme from catalyzing the conversion of HMG-CoA to mevalonic acid, thereby inhibiting cholesterol synthesis .
Biochemical Pathways
The inhibition of HMG-CoA reductase by lovastatin affects the mevalonate pathway , which is responsible for the production of cholesterol and other important biomolecules . By blocking this pathway, lovastatin reduces the levels of low-density lipoprotein (LDL) cholesterol, sometimes referred to as “bad cholesterol”, and very low-density lipoprotein (VLDL) .
Pharmacokinetics
Lovastatin is absorbed orally with a variable range of bioavailability . It is metabolized in the liver by CYP3A4 isoenzymes , which can lead to significant drug interactions . The influence of intake at the time of administration is very important to achieve an adequate therapeutic effect .
Result of Action
The primary result of lovastatin’s action is a reduction in LDL cholesterol levels . This reduction in cholesterol levels decreases the risk of cardiovascular diseases like heart attack or stroke . Lovastatin may also exert other effects independent of ldl-c lowering, termed pleiotropic effects .
Action Environment
The effectiveness of lovastatin can be influenced by various environmental factors. For instance, new unexpected environmental stimuli have been identified, which induce the synthesis of lovastatin, like quorum sensing-type molecules and support stimuli . Additionally, the presence of certain molecules like butyrolactone I, oxylipins, and spermidine, or the addition of ROS-generating molecules to increase internal ROS levels in the cell, can affect lovastatin’s action .
Biochemical Analysis
Biochemical Properties
Lovastatin acid interacts with HMG-CoA reductase, a key enzyme in the mevalonate pathway . By competitively inhibiting this enzyme, this compound prevents the conversion of HMG-CoA to mevalonic acid, a critical step in cholesterol biosynthesis . This interaction reduces the production of cholesterol in the liver, leading to a decrease in low-density lipoprotein (LDL) cholesterol levels in the blood .
Cellular Effects
This compound has significant effects on various types of cells and cellular processes. It influences cell function by impacting cell signaling pathways, gene expression, and cellular metabolism . For instance, this compound can induce apoptosis, a form of programmed cell death, in certain types of cells . It also affects the expression of major histocompatibility complex class II (MHC-II) molecules on various cell types .
Molecular Mechanism
This compound exerts its effects at the molecular level through several mechanisms. It binds to HMG-CoA reductase, inhibiting the enzyme’s activity and thereby reducing the production of mevalonic acid . This leads to a decrease in cholesterol synthesis. This compound can also influence gene expression. For example, it has been shown to promote the accumulation of mitochondrial DNA in the cytosol, activating the cGAS-STING pathway and leading to apoptosis .
Temporal Effects in Laboratory Settings
The effects of this compound can change over time in laboratory settings. For instance, the drug’s effectiveness at reducing LDL cholesterol levels can vary depending on the duration of treatment . This compound is also subject to metabolic degradation, which can influence its long-term effects on cellular function .
Dosage Effects in Animal Models
In animal models, the effects of this compound can vary with different dosages. For instance, studies have shown that this compound can significantly reduce methane emissions in goats when administered at certain dosages . Higher dosages may lead to less palatability and potential adverse effects .
Metabolic Pathways
This compound is involved in the mevalonate pathway, where it interacts with the enzyme HMG-CoA reductase . By inhibiting this enzyme, this compound reduces the production of mevalonic acid, a key intermediate in the synthesis of cholesterol . This interaction can also affect metabolic flux and the levels of other metabolites in the pathway .
Transport and Distribution
This compound is transported and distributed within cells and tissues in several ways. It can cross the blood-brain barrier and the placenta . This compound is also highly bound to plasma proteins, largely due to its lipophilicity . This binding can influence the drug’s distribution within the body .
Subcellular Localization
This compound can be found in various subcellular locations. For instance, it has been shown to accumulate in the cytosol of certain cell types . The drug’s activity and function can be influenced by its subcellular localization, as well as by any targeting signals or post-translational modifications that direct it to specific compartments or organelles .
Preparation Methods
Synthetic Routes and Reaction Conditions
Mevinolinic acid is typically obtained through the hydrolysis of mevinolin. The structure and absolute configuration of mevinolin and its open acid form, mevinolinic acid, were determined by a combination of physical techniques . The synthetic route involves the conversion of mevinolin to mevinolinic acid by breaking the lactone ring under acidic or basic conditions .
Industrial Production Methods
Industrial production of mevinolinic acid involves the fermentation of Aspergillus terreus. The fermentation process is optimized by controlling various parameters such as pH, temperature, and nutrient supply. Recent advancements in the regulation of lovastatin biosynthesis, including the use of reactive oxygen species (ROS) and quorum sensing-type molecules, have improved the yield of mevinolinic acid .
Chemical Reactions Analysis
Types of Reactions
Mevinolinic acid undergoes several types of chemical reactions, including:
Oxidation: Mevinolinic acid can be oxidized to form various oxidized derivatives.
Reduction: Reduction reactions can convert mevinolinic acid to its reduced forms.
Substitution: Mevinolinic acid can undergo substitution reactions where functional groups are replaced by other groups.
Common Reagents and Conditions
Common reagents used in the reactions of mevinolinic acid include oxidizing agents like potassium permanganate and reducing agents like sodium borohydride. The reactions are typically carried out under controlled temperature and pH conditions to ensure the desired product formation .
Major Products Formed
The major products formed from the reactions of mevinolinic acid include various oxidized and reduced derivatives, which have different biological activities and applications .
Scientific Research Applications
Mevinolinic acid has a wide range of scientific research applications:
Comparison with Similar Compounds
Similar Compounds
Compactin (ML-236B): Another potent inhibitor of HMG-CoA reductase, similar in structure to mevinolinic acid.
Lovastatin: The lactone form of mevinolinic acid, also a competitive inhibitor of HMG-CoA reductase.
Simvastatin: A semisynthetic derivative of lovastatin with similar inhibitory properties.
Uniqueness
Mevinolinic acid is unique due to its high potency as an HMG-CoA reductase inhibitor, with a Ki value of 0.6 nM compared to 1.4 nM for compactin . This high potency makes it an effective compound for reducing cholesterol synthesis and has significant implications in the development of cholesterol-lowering drugs .
Properties
IUPAC Name |
(3R,5R)-7-[(1S,2S,6R,8S,8aR)-2,6-dimethyl-8-[(2S)-2-methylbutanoyl]oxy-1,2,6,7,8,8a-hexahydronaphthalen-1-yl]-3,5-dihydroxyheptanoic acid | |
---|---|---|
Source | PubChem | |
URL | https://pubchem.ncbi.nlm.nih.gov | |
Description | Data deposited in or computed by PubChem | |
InChI |
InChI=1S/C24H38O6/c1-5-15(3)24(29)30-21-11-14(2)10-17-7-6-16(4)20(23(17)21)9-8-18(25)12-19(26)13-22(27)28/h6-7,10,14-16,18-21,23,25-26H,5,8-9,11-13H2,1-4H3,(H,27,28)/t14-,15-,16-,18+,19+,20-,21-,23-/m0/s1 | |
Source | PubChem | |
URL | https://pubchem.ncbi.nlm.nih.gov | |
Description | Data deposited in or computed by PubChem | |
InChI Key |
QLJODMDSTUBWDW-BXMDZJJMSA-N | |
Source | PubChem | |
URL | https://pubchem.ncbi.nlm.nih.gov | |
Description | Data deposited in or computed by PubChem | |
Canonical SMILES |
CCC(C)C(=O)OC1CC(C=C2C1C(C(C=C2)C)CCC(CC(CC(=O)O)O)O)C | |
Source | PubChem | |
URL | https://pubchem.ncbi.nlm.nih.gov | |
Description | Data deposited in or computed by PubChem | |
Isomeric SMILES |
CC[C@H](C)C(=O)O[C@H]1C[C@H](C=C2[C@H]1[C@H]([C@H](C=C2)C)CC[C@H](C[C@H](CC(=O)O)O)O)C | |
Source | PubChem | |
URL | https://pubchem.ncbi.nlm.nih.gov | |
Description | Data deposited in or computed by PubChem | |
Molecular Formula |
C24H38O6 | |
Source | PubChem | |
URL | https://pubchem.ncbi.nlm.nih.gov | |
Description | Data deposited in or computed by PubChem | |
DSSTOX Substance ID |
DTXSID20873334 | |
Record name | Lovastatin acid | |
Source | EPA DSSTox | |
URL | https://comptox.epa.gov/dashboard/DTXSID20873334 | |
Description | DSSTox provides a high quality public chemistry resource for supporting improved predictive toxicology. | |
Molecular Weight |
422.6 g/mol | |
Source | PubChem | |
URL | https://pubchem.ncbi.nlm.nih.gov | |
Description | Data deposited in or computed by PubChem | |
CAS No. |
75225-51-3 | |
Record name | Lovastatin acid | |
Source | CAS Common Chemistry | |
URL | https://commonchemistry.cas.org/detail?cas_rn=75225-51-3 | |
Description | CAS Common Chemistry is an open community resource for accessing chemical information. Nearly 500,000 chemical substances from CAS REGISTRY cover areas of community interest, including common and frequently regulated chemicals, and those relevant to high school and undergraduate chemistry classes. This chemical information, curated by our expert scientists, is provided in alignment with our mission as a division of the American Chemical Society. | |
Explanation | The data from CAS Common Chemistry is provided under a CC-BY-NC 4.0 license, unless otherwise stated. | |
Record name | Mevinolinic acid | |
Source | ChemIDplus | |
URL | https://pubchem.ncbi.nlm.nih.gov/substance/?source=chemidplus&sourceid=0075225513 | |
Description | ChemIDplus is a free, web search system that provides access to the structure and nomenclature authority files used for the identification of chemical substances cited in National Library of Medicine (NLM) databases, including the TOXNET system. | |
Record name | Mevinolinic acid | |
Source | DrugBank | |
URL | https://www.drugbank.ca/drugs/DB03785 | |
Description | The DrugBank database is a unique bioinformatics and cheminformatics resource that combines detailed drug (i.e. chemical, pharmacological and pharmaceutical) data with comprehensive drug target (i.e. sequence, structure, and pathway) information. | |
Explanation | Creative Common's Attribution-NonCommercial 4.0 International License (http://creativecommons.org/licenses/by-nc/4.0/legalcode) | |
Record name | Lovastatin acid | |
Source | EPA DSSTox | |
URL | https://comptox.epa.gov/dashboard/DTXSID20873334 | |
Description | DSSTox provides a high quality public chemistry resource for supporting improved predictive toxicology. | |
Record name | LOVASTATIN ACID | |
Source | FDA Global Substance Registration System (GSRS) | |
URL | https://gsrs.ncats.nih.gov/ginas/app/beta/substances/5CLV35Y90C | |
Description | The FDA Global Substance Registration System (GSRS) enables the efficient and accurate exchange of information on what substances are in regulated products. Instead of relying on names, which vary across regulatory domains, countries, and regions, the GSRS knowledge base makes it possible for substances to be defined by standardized, scientific descriptions. | |
Explanation | Unless otherwise noted, the contents of the FDA website (www.fda.gov), both text and graphics, are not copyrighted. They are in the public domain and may be republished, reprinted and otherwise used freely by anyone without the need to obtain permission from FDA. Credit to the U.S. Food and Drug Administration as the source is appreciated but not required. | |
Retrosynthesis Analysis
AI-Powered Synthesis Planning: Our tool employs the Template_relevance Pistachio, Template_relevance Bkms_metabolic, Template_relevance Pistachio_ringbreaker, Template_relevance Reaxys, Template_relevance Reaxys_biocatalysis model, leveraging a vast database of chemical reactions to predict feasible synthetic routes.
One-Step Synthesis Focus: Specifically designed for one-step synthesis, it provides concise and direct routes for your target compounds, streamlining the synthesis process.
Accurate Predictions: Utilizing the extensive PISTACHIO, BKMS_METABOLIC, PISTACHIO_RINGBREAKER, REAXYS, REAXYS_BIOCATALYSIS database, our tool offers high-accuracy predictions, reflecting the latest in chemical research and data.
Strategy Settings
Precursor scoring | Relevance Heuristic |
---|---|
Min. plausibility | 0.01 |
Model | Template_relevance |
Template Set | Pistachio/Bkms_metabolic/Pistachio_ringbreaker/Reaxys/Reaxys_biocatalysis |
Top-N result to add to graph | 6 |
Feasible Synthetic Routes
Disclaimer and Information on In-Vitro Research Products
Please be aware that all articles and product information presented on BenchChem are intended solely for informational purposes. The products available for purchase on BenchChem are specifically designed for in-vitro studies, which are conducted outside of living organisms. In-vitro studies, derived from the Latin term "in glass," involve experiments performed in controlled laboratory settings using cells or tissues. It is important to note that these products are not categorized as medicines or drugs, and they have not received approval from the FDA for the prevention, treatment, or cure of any medical condition, ailment, or disease. We must emphasize that any form of bodily introduction of these products into humans or animals is strictly prohibited by law. It is essential to adhere to these guidelines to ensure compliance with legal and ethical standards in research and experimentation.