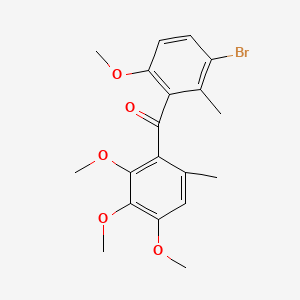
Metrafenone
Overview
Description
Metrafenone is a benzophenone fungicide known for its protectant and curative properties. It is primarily used to control powdery mildew on various crops, including grapes, wheat, barley, and other cereals. This compound inhibits the growth of mycelium on the leaf surface, leaf penetration, the formation of haustoria, and sporulation .
Mechanism of Action
Target of Action
Metrafenone is a benzophenone fungicide that primarily targets powdery mildew fungi . It is particularly effective against Erysiphe graminis and Pseudocercosporella herpotrichoides . The primary targets of this compound are the hyphae of these fungi, where it interferes with their growth and development .
Mode of Action
This compound operates by interfering with the morphogenesis of the fungal hyphae . It disrupts the formation of the apical actin cap and apical vesicle transport, which are crucial for the growth and development of the hyphae . This disruption leads to a weakening of the cell wall at the hyphal tips, causing swelling, bursting, and collapse of the hyphal tips . This results in the release of globules of cytoplasm and inhibits the germination of spores .
Biochemical Pathways
It is suggested that this compound likely disturbs a pathway regulating the organization of the actin cytoskeleton . This disruption affects the polarized growth of the hyphae and the establishment and maintenance of cell polarity .
Pharmacokinetics
It is known that this compound is rapidly absorbed and widely distributed in the body . It is extensively metabolized, with most of the metabolites excreted as glucuronic acid conjugates in bile and urine .
Result of Action
The action of this compound results in significant molecular and cellular effects. It causes abnormalities in hyphal growth and malformation of conidiophores . These effects are associated with irregular septation, multinucleate cells, and delocalization of actin . This compound also strongly reduces sporulation .
Action Environment
The efficacy of this compound can be influenced by environmental factors. It is known that this compound is used in various environments for the control of diseases on cereals and other crops .
Biochemical Analysis
Biochemical Properties
Metrafenone interferes with hyphal morphogenesis . It inhibits the growth of the mycelium on the leaf surface, leaf penetration, the formation of haustoria, and sporulation . This compound is extensively metabolized, with most of the labelled products excreted as glucuronic acid conjugates in bile and urine .
Cellular Effects
This compound has been observed to cause disruption of the apical actin cap and apical vesicle transport, as well as weakening of the cell wall at hyphal tips . It also induces swelling, bursting, and collapse of hyphal tips, resulting in the release of globules of cytoplasm .
Molecular Mechanism
It has been suggested that this compound likely disturbs a pathway regulating the organization of the actin cytoskeleton .
Temporal Effects in Laboratory Settings
Over time, this compound has been observed to continuously decrease the level of extractable radioactivity in soil samples, suggesting the formation of bound residues . The labelled material was relatively rapidly excreted into the gastrointestinal tract via the bile (85–90%), resulting in extensive excretion via faeces .
Dosage Effects in Animal Models
In rats, absorption of this compound was rapid and complete (> 88%) at the low dose of 10 mg/kg body weight but was limited to 15–20% at the high dose of 1000 mg/kg body weight, suggesting saturation of the absorption processes .
Metabolic Pathways
This compound undergoes several transformation steps including: O-demethylation of the aromatic methoxy group(s) followed by mono-O-glycosidation; hydroxylation of the bromophenyl ring; and hydroxylation of the methyl substituent to hydroxymethyl followed by O-glycosidation or further oxidation to aldehyde or lactone .
Transport and Distribution
Following oral administration in rats, this compound was widely distributed in the body, with the highest residue levels found mainly in the gastrointestinal tract, liver, and fat .
Subcellular Localization
Given its role as a fungicide and its observed effects on hyphal tips and the actin cytoskeleton , it can be inferred that this compound likely localizes to areas of active growth in the cell.
Preparation Methods
The synthesis of metrafenone involves several steps, including diazotization, hydrolysis, etherification, selective oxidation, positioning oxidation, bromination, acylating chlorination, methylation, methoxylation, and Friedel-Crafts acylation. The process starts with the preparation of a crude product of this compound (3’-bromo-2,3,4,6’-tetramethoxy-2’,6-dimethyl benzophenone) and ends with refining to obtain the target product .
Chemical Reactions Analysis
Metrafenone undergoes various chemical reactions, including:
Oxidation: this compound can be oxidized, leading to the formation of different oxidation products.
Reduction: Reduction reactions can modify the functional groups present in this compound.
Substitution: this compound can undergo substitution reactions, where functional groups are replaced by other groups.
Common Reagents and Conditions: Typical reagents include oxidizing agents, reducing agents, and various catalysts. Reaction conditions vary depending on the desired product.
Major Products: The major products formed from these reactions include various derivatives of this compound, which can be used for different applications .
Scientific Research Applications
Metrafenone has a wide range of scientific research applications:
Chemistry: Used as a model compound for studying benzophenone derivatives and their reactions.
Biology: Investigated for its effects on fungal pathogens and its potential use in controlling fungal diseases in crops.
Medicine: Explored for its potential antifungal properties and its mechanism of action against fungal infections.
Industry: Utilized in the agricultural industry as a fungicide to protect crops from powdery mildew and other fungal diseases .
Comparison with Similar Compounds
Metrafenone is unique among benzophenone fungicides due to its specific mode of action and its effectiveness against powdery mildew. Similar compounds include:
Boscalid: Another fungicide used to control powdery mildew, but with a different mode of action.
Kresoxim-methyl: A fungicide that also targets powdery mildew but works by inhibiting mitochondrial respiration.
Pyriofenone: A fungicide similar to this compound, but with a pyridine ring and different substitution patterns
This compound stands out due to its unique mechanism of action and its broad-spectrum activity against various fungal pathogens.
Properties
IUPAC Name |
(3-bromo-6-methoxy-2-methylphenyl)-(2,3,4-trimethoxy-6-methylphenyl)methanone | |
---|---|---|
Source | PubChem | |
URL | https://pubchem.ncbi.nlm.nih.gov | |
Description | Data deposited in or computed by PubChem | |
InChI |
InChI=1S/C19H21BrO5/c1-10-9-14(23-4)18(24-5)19(25-6)15(10)17(21)16-11(2)12(20)7-8-13(16)22-3/h7-9H,1-6H3 | |
Source | PubChem | |
URL | https://pubchem.ncbi.nlm.nih.gov | |
Description | Data deposited in or computed by PubChem | |
InChI Key |
AMSPWOYQQAWRRM-UHFFFAOYSA-N | |
Source | PubChem | |
URL | https://pubchem.ncbi.nlm.nih.gov | |
Description | Data deposited in or computed by PubChem | |
Canonical SMILES |
CC1=CC(=C(C(=C1C(=O)C2=C(C=CC(=C2C)Br)OC)OC)OC)OC | |
Source | PubChem | |
URL | https://pubchem.ncbi.nlm.nih.gov | |
Description | Data deposited in or computed by PubChem | |
Molecular Formula |
C19H21BrO5 | |
Source | PubChem | |
URL | https://pubchem.ncbi.nlm.nih.gov | |
Description | Data deposited in or computed by PubChem | |
DSSTOX Substance ID |
DTXSID9058020 | |
Record name | Metrafenone | |
Source | EPA DSSTox | |
URL | https://comptox.epa.gov/dashboard/DTXSID9058020 | |
Description | DSSTox provides a high quality public chemistry resource for supporting improved predictive toxicology. | |
Molecular Weight |
409.3 g/mol | |
Source | PubChem | |
URL | https://pubchem.ncbi.nlm.nih.gov | |
Description | Data deposited in or computed by PubChem | |
CAS No. |
220899-03-6 | |
Record name | Metrafenone | |
Source | CAS Common Chemistry | |
URL | https://commonchemistry.cas.org/detail?cas_rn=220899-03-6 | |
Description | CAS Common Chemistry is an open community resource for accessing chemical information. Nearly 500,000 chemical substances from CAS REGISTRY cover areas of community interest, including common and frequently regulated chemicals, and those relevant to high school and undergraduate chemistry classes. This chemical information, curated by our expert scientists, is provided in alignment with our mission as a division of the American Chemical Society. | |
Explanation | The data from CAS Common Chemistry is provided under a CC-BY-NC 4.0 license, unless otherwise stated. | |
Record name | Metrafenone [ISO] | |
Source | ChemIDplus | |
URL | https://pubchem.ncbi.nlm.nih.gov/substance/?source=chemidplus&sourceid=0220899036 | |
Description | ChemIDplus is a free, web search system that provides access to the structure and nomenclature authority files used for the identification of chemical substances cited in National Library of Medicine (NLM) databases, including the TOXNET system. | |
Record name | Metrafenone | |
Source | EPA DSSTox | |
URL | https://comptox.epa.gov/dashboard/DTXSID9058020 | |
Description | DSSTox provides a high quality public chemistry resource for supporting improved predictive toxicology. | |
Record name | METRAFENONE | |
Source | FDA Global Substance Registration System (GSRS) | |
URL | https://gsrs.ncats.nih.gov/ginas/app/beta/substances/S04CWW41HM | |
Description | The FDA Global Substance Registration System (GSRS) enables the efficient and accurate exchange of information on what substances are in regulated products. Instead of relying on names, which vary across regulatory domains, countries, and regions, the GSRS knowledge base makes it possible for substances to be defined by standardized, scientific descriptions. | |
Explanation | Unless otherwise noted, the contents of the FDA website (www.fda.gov), both text and graphics, are not copyrighted. They are in the public domain and may be republished, reprinted and otherwise used freely by anyone without the need to obtain permission from FDA. Credit to the U.S. Food and Drug Administration as the source is appreciated but not required. | |
Synthesis routes and methods
Procedure details
Retrosynthesis Analysis
AI-Powered Synthesis Planning: Our tool employs the Template_relevance Pistachio, Template_relevance Bkms_metabolic, Template_relevance Pistachio_ringbreaker, Template_relevance Reaxys, Template_relevance Reaxys_biocatalysis model, leveraging a vast database of chemical reactions to predict feasible synthetic routes.
One-Step Synthesis Focus: Specifically designed for one-step synthesis, it provides concise and direct routes for your target compounds, streamlining the synthesis process.
Accurate Predictions: Utilizing the extensive PISTACHIO, BKMS_METABOLIC, PISTACHIO_RINGBREAKER, REAXYS, REAXYS_BIOCATALYSIS database, our tool offers high-accuracy predictions, reflecting the latest in chemical research and data.
Strategy Settings
Precursor scoring | Relevance Heuristic |
---|---|
Min. plausibility | 0.01 |
Model | Template_relevance |
Template Set | Pistachio/Bkms_metabolic/Pistachio_ringbreaker/Reaxys/Reaxys_biocatalysis |
Top-N result to add to graph | 6 |
Feasible Synthetic Routes
Q1: What is known about the mode of action of metrafenone?
A1: Although the precise molecular target of this compound is still under investigation, research suggests it disrupts fungal development by interfering with hyphal morphogenesis, polarized hyphal growth, and cell polarity. [, ] This disruption manifests as swelling, bursting, and collapse of hyphal tips, along with abnormal appressoria formation and hyperbranching. []
Q2: How does this compound affect the actin cytoskeleton in fungi?
A2: Studies using Blumeria graminis, a powdery mildew fungus, revealed that this compound disrupts the apical actin cap, hindering apical vesicle transport essential for hyphal growth. [] This disruption weakens the cell wall at hyphal tips, contributing to the morphological abnormalities observed. []
Q3: Does this compound impact fungal sporulation?
A3: Yes, this compound significantly reduces sporulation in powdery mildew fungi. [] It induces malformation of conidiophores, leading to irregular septation, multinucleate cells, and delocalization of actin, ultimately hindering spore production. []
Q4: What is the molecular formula and weight of this compound?
A4: this compound has the molecular formula C19H20O4 and a molecular weight of 312.36 g/mol. []
Q5: Does the application of this compound impact the aroma profile of wines made from treated grapes?
A5: Research has shown that this compound application can influence the volatile composition of wines. For instance, in Graciano wines, this compound treatment increased the concentration of varietal volatile compounds and aldehydes while decreasing acetates and aromatic alcohols. [] Tempranillo wines also exhibited aroma profile modifications depending on the specific fungicide used in conjunction with this compound. []
Q6: Has resistance to this compound been reported in powdery mildew populations?
A6: Yes, this compound resistance has been identified in Erysiphe necator, the causal agent of grape powdery mildew, in Italy. [] This resistance was observed in field populations and confirmed through laboratory sensitivity testing of monoconidial isolates. []
Q7: Does cross-resistance exist between this compound and other fungicides?
A7: Cross-resistance has been observed between this compound and pyriofenone, another benzophenone fungicide. [] This cross-resistance suggests that these fungicides may share a similar mode of action or target site, although further research is needed to confirm this. []
Q8: What analytical techniques are commonly employed for the detection and quantification of this compound residues?
A8: Liquid chromatography coupled with tandem mass spectrometry (LC-MS/MS) is widely utilized for the analysis of this compound residues in various matrices, including grapes, wine, and cherry tomatoes. [, ] This technique offers high sensitivity and selectivity, enabling accurate quantification of this compound residues even at low concentrations. []
Q9: Have analytical methods for this compound residue analysis been validated?
A9: Yes, validated analytical methods for this compound residue analysis have been established, adhering to guidelines set forth by regulatory bodies such as SANCO. [] These methods demonstrate acceptable accuracy, precision, and specificity, ensuring reliable quantification of this compound residues in various matrices. []
Q10: What is the environmental fate of this compound?
A10: The environmental fate and degradation of this compound have been investigated, revealing its persistence in soil and potential for leaching into groundwater. [] Further research is needed to fully understand its long-term ecological impacts and develop mitigation strategies. []
Disclaimer and Information on In-Vitro Research Products
Please be aware that all articles and product information presented on BenchChem are intended solely for informational purposes. The products available for purchase on BenchChem are specifically designed for in-vitro studies, which are conducted outside of living organisms. In-vitro studies, derived from the Latin term "in glass," involve experiments performed in controlled laboratory settings using cells or tissues. It is important to note that these products are not categorized as medicines or drugs, and they have not received approval from the FDA for the prevention, treatment, or cure of any medical condition, ailment, or disease. We must emphasize that any form of bodily introduction of these products into humans or animals is strictly prohibited by law. It is essential to adhere to these guidelines to ensure compliance with legal and ethical standards in research and experimentation.