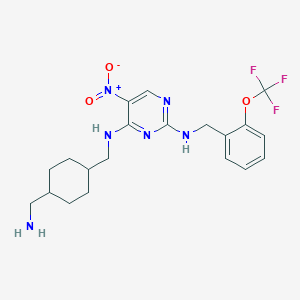
PKC-theta inhibitor
Overview
Description
PKC-theta inhibitor is a useful research compound. Its molecular formula is C20H25F3N6O3 and its molecular weight is 454.4 g/mol. The purity is usually >98% (or refer to the Certificate of Analysis).
The exact mass of the compound this compound is 454.194 and the complexity rating of the compound is unknown. The solubility of this chemical has been described as Soluble in DMSO. The storage condition is described as Dry, dark and at 0 - 4 C for short term (days to weeks) or -20 C for long term (months to years)..
BenchChem offers high-quality this compound suitable for many research applications. Different packaging options are available to accommodate customers' requirements. Please inquire for more information about this compound including the price, delivery time, and more detailed information at info@benchchem.com.
Mechanism of Action
Target of Action
The primary target of PKC-theta inhibitors is Protein Kinase C theta (PKCθ) . PKCθ is a serine/threonine kinase that belongs to the novel PKC subfamily . It plays a critical role in several essential cellular processes such as survival, proliferation, and differentiation . PKCθ is also a key player in carcinogenesis, exerting both promotive and suppressive effects on tumor cell growth and metastasis .
Mode of Action
PKC-theta inhibitors, like Compound 20 (C20), selectively inhibit the nuclear translocation of PKCθ . PKCθ inhibitors block the catalytic activity of PKC enzymes, such as PKCα, PKCβ, PKCθ, and PKCδ . This inhibition leads to the suppression of pathways like PKC/ERK1/2 and PKC/NF-κB .
Biochemical Pathways
PKCθ is involved in multiple signal transduction pathways that turn environmental cues into cellular actions . PKCθ controls T cell activation, survival, and differentiation . Inhibition of PKCθ leads to a significant reduction in the number of immune cells infiltrating the heart, as well as necrosis and fibrosis .
Pharmacokinetics
One study showed that pkcθ inhibitor 1 inhibits il-2 production in vivo with an ic50 of 019 μM . This suggests that PKCθ inhibitors can effectively penetrate tissues and reach their target cells.
Result of Action
The inhibition of PKCθ leads to a significant reduction in the number of immune cells infiltrating the heart, as well as necrosis and fibrosis . This results in a reduction of symptoms in a mouse model of multiple sclerosis . In cancer, high PKCθ expression leads to aberrant cell proliferation, migration, and invasion, resulting in a malignant phenotype . The inhibition of PKCθ can reduce these effects .
Biochemical Analysis
Biochemical Properties
PKC-theta inhibitors interact with various enzymes, proteins, and biomolecules to exert their effects. One such inhibitor, EXS4318, has shown high selectivity and potency against PKC-theta. This inhibitor binds to the catalytic domain of PKC-theta, preventing its activation and subsequent phosphorylation of downstream targets. The interaction between PKC-theta inhibitors and PKC-theta is highly specific, minimizing off-target effects and ensuring effective modulation of T-cell function .
Cellular Effects
PKC-theta inhibitors have significant effects on various cell types and cellular processes. In T-cells, these inhibitors downregulate the production of interleukin-2 (IL-2) by inhibiting the activation of transcription factors such as nuclear factor-kappa B (NF-kappa B) and nuclear factor of activated T-cells (NF-AT). This results in reduced T-cell proliferation and activation, which is beneficial in treating autoimmune diseases . Additionally, PKC-theta inhibitors can modulate the balance between regulatory T-cells (Tregs) and effector T-cells (Teffs), further influencing immune responses .
Molecular Mechanism
The molecular mechanism of PKC-theta inhibitors involves binding to the catalytic domain of PKC-theta, preventing its activation by diacylglycerol and phosphatidylserine. This inhibition blocks the phosphorylation of downstream targets, such as CARMA/BCL10/MALT (CBM) signaling complex, which is essential for T-cell activation . By inhibiting PKC-theta, these inhibitors reduce the activation of transcription factors NF-kappa B and NF-AT, leading to decreased IL-2 production and T-cell proliferation .
Temporal Effects in Laboratory Settings
In laboratory settings, the effects of PKC-theta inhibitors can vary over time. Studies have shown that these inhibitors maintain their stability and potency over extended periods, ensuring consistent modulation of T-cell function . Long-term exposure to PKC-theta inhibitors has been observed to result in sustained suppression of T-cell activation and proliferation, with minimal degradation of the compound .
Dosage Effects in Animal Models
The effects of PKC-theta inhibitors in animal models vary with different dosages. At low doses, these inhibitors effectively reduce T-cell activation and proliferation without causing significant adverse effects . At higher doses, PKC-theta inhibitors may exhibit toxic effects, such as impaired immune responses and increased susceptibility to infections . It is crucial to determine the optimal dosage to balance therapeutic efficacy and safety.
Metabolic Pathways
PKC-theta inhibitors are involved in various metabolic pathways, including the inhibition of glycolysis and the tricarboxylic acid (TCA) cycle. These inhibitors also affect the pentose phosphate pathway, fatty acid oxidation, and cholesterol transport . By modulating these metabolic pathways, PKC-theta inhibitors can influence cellular energy production and overall metabolic flux .
Transport and Distribution
PKC-theta inhibitors are transported and distributed within cells and tissues through specific transporters and binding proteins. These inhibitors localize primarily in T-cells, where they exert their effects on T-cell activation and function . The distribution of PKC-theta inhibitors within tissues is influenced by their binding affinity to PKC-theta and other cellular components .
Subcellular Localization
The subcellular localization of PKC-theta inhibitors is primarily within the cytoplasm and at the immunological synapse, where PKC-theta is activated. These inhibitors target the catalytic domain of PKC-theta, preventing its translocation to the plasma membrane and subsequent activation . By inhibiting PKC-theta at these specific subcellular locations, these inhibitors effectively modulate T-cell activation and immune responses .
Properties
IUPAC Name |
4-N-[[4-(aminomethyl)cyclohexyl]methyl]-5-nitro-2-N-[[2-(trifluoromethoxy)phenyl]methyl]pyrimidine-2,4-diamine | |
---|---|---|
Source | PubChem | |
URL | https://pubchem.ncbi.nlm.nih.gov | |
Description | Data deposited in or computed by PubChem | |
InChI |
InChI=1S/C20H25F3N6O3/c21-20(22,23)32-17-4-2-1-3-15(17)11-26-19-27-12-16(29(30)31)18(28-19)25-10-14-7-5-13(9-24)6-8-14/h1-4,12-14H,5-11,24H2,(H2,25,26,27,28) | |
Source | PubChem | |
URL | https://pubchem.ncbi.nlm.nih.gov | |
Description | Data deposited in or computed by PubChem | |
InChI Key |
HKOWATVSFKRXRW-UHFFFAOYSA-N | |
Source | PubChem | |
URL | https://pubchem.ncbi.nlm.nih.gov | |
Description | Data deposited in or computed by PubChem | |
Canonical SMILES |
C1CC(CCC1CN)CNC2=NC(=NC=C2[N+](=O)[O-])NCC3=CC=CC=C3OC(F)(F)F | |
Source | PubChem | |
URL | https://pubchem.ncbi.nlm.nih.gov | |
Description | Data deposited in or computed by PubChem | |
Molecular Formula |
C20H25F3N6O3 | |
Source | PubChem | |
URL | https://pubchem.ncbi.nlm.nih.gov | |
Description | Data deposited in or computed by PubChem | |
Molecular Weight |
454.4 g/mol | |
Source | PubChem | |
URL | https://pubchem.ncbi.nlm.nih.gov | |
Description | Data deposited in or computed by PubChem | |
Retrosynthesis Analysis
AI-Powered Synthesis Planning: Our tool employs the Template_relevance Pistachio, Template_relevance Bkms_metabolic, Template_relevance Pistachio_ringbreaker, Template_relevance Reaxys, Template_relevance Reaxys_biocatalysis model, leveraging a vast database of chemical reactions to predict feasible synthetic routes.
One-Step Synthesis Focus: Specifically designed for one-step synthesis, it provides concise and direct routes for your target compounds, streamlining the synthesis process.
Accurate Predictions: Utilizing the extensive PISTACHIO, BKMS_METABOLIC, PISTACHIO_RINGBREAKER, REAXYS, REAXYS_BIOCATALYSIS database, our tool offers high-accuracy predictions, reflecting the latest in chemical research and data.
Strategy Settings
Precursor scoring | Relevance Heuristic |
---|---|
Min. plausibility | 0.01 |
Model | Template_relevance |
Template Set | Pistachio/Bkms_metabolic/Pistachio_ringbreaker/Reaxys/Reaxys_biocatalysis |
Top-N result to add to graph | 6 |
Feasible Synthetic Routes
Disclaimer and Information on In-Vitro Research Products
Please be aware that all articles and product information presented on BenchChem are intended solely for informational purposes. The products available for purchase on BenchChem are specifically designed for in-vitro studies, which are conducted outside of living organisms. In-vitro studies, derived from the Latin term "in glass," involve experiments performed in controlled laboratory settings using cells or tissues. It is important to note that these products are not categorized as medicines or drugs, and they have not received approval from the FDA for the prevention, treatment, or cure of any medical condition, ailment, or disease. We must emphasize that any form of bodily introduction of these products into humans or animals is strictly prohibited by law. It is essential to adhere to these guidelines to ensure compliance with legal and ethical standards in research and experimentation.