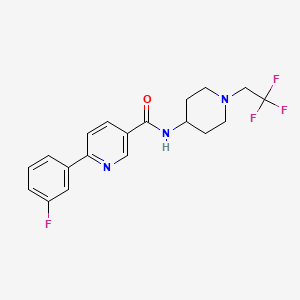
HPGDS inhibitor 1
Overview
Description
HPGDS inhibitor 1 is a potent, selective, and orally active inhibitor of hematopoietic prostaglandin D synthase (HPGDS). This enzyme is involved in the production of prostaglandin D2, which plays a role in various physiological processes, including inflammation, allergic reactions, and sleep regulation . This compound has been investigated as a potential anti-inflammatory agent .
Mechanism of Action
Target of Action
HPGDS inhibitor 1 is a potent, selective, and orally active inhibitor of Hematopoietic Prostaglandin D Synthase (HPGDS) . HPGDS is an enzyme that catalyzes the conversion of PGH2 to PGD2 , a prostaglandin involved in various physiological processes including inflammation and allergic reactions .
Mode of Action
This compound interacts with its target, HPGDS, by binding to the enzyme and inhibiting its activity . This inhibition prevents the conversion of PGH2 to PGD2, thereby controlling the level of PGD2 in the body .
Biochemical Pathways
The primary biochemical pathway affected by this compound is the arachidonic acid/COX pathway . By inhibiting HPGDS, the compound prevents the conversion of PGH2 to PGD2, disrupting the normal flow of this pathway . This can have downstream effects on various physiological processes, including inflammation and allergic reactions .
Result of Action
The primary result of this compound’s action is the reduction of PGD2 levels in the body . This can lead to a decrease in inflammation and allergic reactions, as PGD2 plays a significant role in these processes . For example, this compound has been shown to control the decline in motor function in Duchenne muscular dystrophy (DMD) patients by inhibiting HPGDS, which exacerbates the inflammatory response in DMD patients’ muscles .
Action Environment
The efficacy and stability of this compound can be influenced by various environmental factors. For instance, the presence of other molecules in the body that can bind to HPGDS could potentially affect the inhibitor’s ability to interact with its target . Additionally, factors such as pH and temperature could potentially influence the stability and activity of the compound.
Biochemical Analysis
Biochemical Properties
HPGDS inhibitor 1 interacts with the enzyme Hematopoietic Prostaglandin D Synthase (HPGDS), inhibiting its activity . The IC50 values in enzyme and cellular assays are 0.6 nM and 32 nM, respectively . Notably, this compound does not inhibit human L-PGDS, mPGES, COX-1, COX-2, or 5-LOX .
Cellular Effects
This compound has significant effects on various types of cells and cellular processes. It has been shown to reduce glutathione (GSH) levels in glioblastoma multiforme (GBM) cell lines, leading to the accumulation of intracellular 4-HNE, a byproduct of lipid peroxidation .
Molecular Mechanism
The molecular mechanism of this compound involves its binding and inhibition of the HPGDS enzyme . This inhibition disrupts the cellular redox balance and inhibits the activation of JNK signaling . It also prevents the conversion of PGH2 to PGD2, a prostaglandin involved in various physiological processes .
Dosage Effects in Animal Models
In animal models, the effects of this compound vary with different dosages. For instance, in a study using the mdx mouse model of Duchenne muscular dystrophy (DMD), it was found that hindlimb grip strength was two-fold greater in the this compound-treated mdx group, compared to untreated mdx mice .
Metabolic Pathways
This compound is involved in the arachidonic acid/COX pathway, acting downstream to catalyze the isomerization of PGH2 to PGD2 . This makes it an interesting target to treat allergic inflammation .
Subcellular Localization
The subcellular localization of this compound is not explicitly documented. The HPGDS enzyme, which this compound targets, is localized to the vesicles and cytosol . Therefore, it can be inferred that this compound may also localize to these subcellular compartments to exert its inhibitory effects.
Preparation Methods
The preparation of HPGDS inhibitor 1 involves synthetic routes and reaction conditions that ensure high purity and efficacy. The compound is synthesized through a series of chemical reactions, including the formation of key intermediates and final product purification. Industrial production methods focus on optimizing yield and scalability while maintaining the compound’s bioactivity .
Chemical Reactions Analysis
HPGDS inhibitor 1 undergoes various chemical reactions, including:
Oxidation: This reaction involves the addition of oxygen or the removal of hydrogen, often using oxidizing agents.
Reduction: This reaction involves the addition of hydrogen or the removal of oxygen, typically using reducing agents.
Substitution: This reaction involves the replacement of one functional group with another, often using nucleophiles or electrophiles.
Common reagents and conditions used in these reactions include oxidizing agents like potassium permanganate, reducing agents like sodium borohydride, and nucleophiles like hydroxide ions. The major products formed from these reactions depend on the specific conditions and reagents used .
Scientific Research Applications
HPGDS inhibitor 1 has a wide range of scientific research applications, including:
Chemistry: Used as a tool to study the role of prostaglandin D2 in various chemical reactions and pathways.
Biology: Investigated for its effects on cellular processes and signaling pathways involving prostaglandin D2.
Medicine: Explored as a potential therapeutic agent for conditions involving inflammation, allergic reactions, and sleep disorders.
Industry: Utilized in the development of new drugs and therapeutic agents targeting prostaglandin D2 pathways
Comparison with Similar Compounds
HPGDS inhibitor 1 is unique in its selectivity and potency compared to other similar compounds. Some similar compounds include:
TFC-007: Another potent inhibitor of hematopoietic prostaglandin D synthase with similar applications.
TAS-204: A compound with a good range of interaction with human hematopoietic prostaglandin D synthase.
This compound stands out due to its high selectivity and oral bioavailability, making it a promising candidate for further research and development .
Properties
IUPAC Name |
6-(3-fluorophenyl)-N-[1-(2,2,2-trifluoroethyl)piperidin-4-yl]pyridine-3-carboxamide | |
---|---|---|
Source | PubChem | |
URL | https://pubchem.ncbi.nlm.nih.gov | |
Description | Data deposited in or computed by PubChem | |
InChI |
InChI=1S/C19H19F4N3O/c20-15-3-1-2-13(10-15)17-5-4-14(11-24-17)18(27)25-16-6-8-26(9-7-16)12-19(21,22)23/h1-5,10-11,16H,6-9,12H2,(H,25,27) | |
Source | PubChem | |
URL | https://pubchem.ncbi.nlm.nih.gov | |
Description | Data deposited in or computed by PubChem | |
InChI Key |
LPUCBGGXXIUBAZ-UHFFFAOYSA-N | |
Source | PubChem | |
URL | https://pubchem.ncbi.nlm.nih.gov | |
Description | Data deposited in or computed by PubChem | |
Canonical SMILES |
C1CN(CCC1NC(=O)C2=CN=C(C=C2)C3=CC(=CC=C3)F)CC(F)(F)F | |
Source | PubChem | |
URL | https://pubchem.ncbi.nlm.nih.gov | |
Description | Data deposited in or computed by PubChem | |
Molecular Formula |
C19H19F4N3O | |
Source | PubChem | |
URL | https://pubchem.ncbi.nlm.nih.gov | |
Description | Data deposited in or computed by PubChem | |
Molecular Weight |
381.4 g/mol | |
Source | PubChem | |
URL | https://pubchem.ncbi.nlm.nih.gov | |
Description | Data deposited in or computed by PubChem | |
Synthesis routes and methods
Procedure details
Retrosynthesis Analysis
AI-Powered Synthesis Planning: Our tool employs the Template_relevance Pistachio, Template_relevance Bkms_metabolic, Template_relevance Pistachio_ringbreaker, Template_relevance Reaxys, Template_relevance Reaxys_biocatalysis model, leveraging a vast database of chemical reactions to predict feasible synthetic routes.
One-Step Synthesis Focus: Specifically designed for one-step synthesis, it provides concise and direct routes for your target compounds, streamlining the synthesis process.
Accurate Predictions: Utilizing the extensive PISTACHIO, BKMS_METABOLIC, PISTACHIO_RINGBREAKER, REAXYS, REAXYS_BIOCATALYSIS database, our tool offers high-accuracy predictions, reflecting the latest in chemical research and data.
Strategy Settings
Precursor scoring | Relevance Heuristic |
---|---|
Min. plausibility | 0.01 |
Model | Template_relevance |
Template Set | Pistachio/Bkms_metabolic/Pistachio_ringbreaker/Reaxys/Reaxys_biocatalysis |
Top-N result to add to graph | 6 |
Feasible Synthetic Routes
Disclaimer and Information on In-Vitro Research Products
Please be aware that all articles and product information presented on BenchChem are intended solely for informational purposes. The products available for purchase on BenchChem are specifically designed for in-vitro studies, which are conducted outside of living organisms. In-vitro studies, derived from the Latin term "in glass," involve experiments performed in controlled laboratory settings using cells or tissues. It is important to note that these products are not categorized as medicines or drugs, and they have not received approval from the FDA for the prevention, treatment, or cure of any medical condition, ailment, or disease. We must emphasize that any form of bodily introduction of these products into humans or animals is strictly prohibited by law. It is essential to adhere to these guidelines to ensure compliance with legal and ethical standards in research and experimentation.