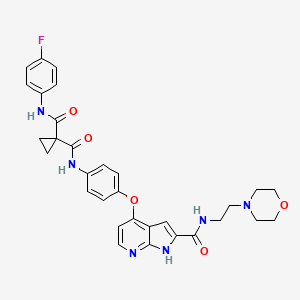
Tyrosine kinase inhibitor
Overview
Description
Tyrosine kinase inhibitors (TKIs) are small-molecule therapeutics designed to block the enzymatic activity of tyrosine kinases, which catalyze the transfer of phosphate groups from ATP to tyrosine residues on target proteins. These kinases regulate critical cellular processes, including proliferation, differentiation, and apoptosis . Dysregulation of tyrosine kinases, due to mutations or overexpression, is implicated in cancers and neurodegenerative disorders, making TKIs pivotal in targeted therapy . TKIs are classified into reversible (competitive ATP-binding site inhibitors) and irreversible (covalent binders) types, with selectivity varying across generations of drugs .
Preparation Methods
Synthetic Routes and Reaction Conditions: One method for preparing tyrosine kinase inhibitors involves using 1,1-cyclopropane dicarboxylic acid diester as a raw material. This compound is converted into 1-((4-((6,7-dimethoxy quinoline-4-yl)oxy)phenyl)carbamoyl)cyclopropane formic ether, which is then hydrolyzed and reacted with p-fluoro aniline to produce Cabozantinib. The reaction conditions are mild, and the synthesis cost is relatively low, making it suitable for industrial production .
Industrial Production Methods: Industrial production of tyrosine kinase inhibitors often involves mixed-mode solid-phase extraction (SPE) for bioanalytical sample preparation. This method achieves high recovery and selectivity of several small molecule oncology therapeutic drugs from plasma using mixed-mode SPE sorbents .
Chemical Reactions Analysis
Types of Reactions: Tyrosine kinase inhibitors undergo various chemical reactions, including:
Oxidation: Involves the addition of oxygen or the removal of hydrogen.
Reduction: Involves the addition of hydrogen or the removal of oxygen.
Substitution: Involves the replacement of one atom or group of atoms with another.
Common Reagents and Conditions: Common reagents used in these reactions include formic acid, methanol, and various organic solvents. Conditions often involve controlled temperatures and pH levels to ensure optimal reaction efficiency.
Major Products: The major products formed from these reactions are typically the active pharmaceutical ingredients (APIs) that inhibit specific tyrosine kinases involved in cancer cell proliferation.
Scientific Research Applications
Classification of Tyrosine Kinase Inhibitors
- Receptor Tyrosine Kinase Inhibitors : Target transmembrane receptors such as epidermal growth factor receptor (EGFR) and vascular endothelial growth factor receptor (VEGFR).
- Non-Receptor Tyrosine Kinase Inhibitors : Act on cytoplasmic kinases involved in signaling pathways.
- Dual-Specificity Kinase Inhibitors : Affect both serine/threonine and tyrosine phosphorylation.
Cancer Treatment
TKIs have revolutionized the treatment landscape for various malignancies:
- Chronic Myelogenous Leukemia (CML) : Imatinib was the first TKI approved for CML, targeting the BCR-ABL fusion protein. Its introduction has significantly improved patient outcomes .
- Non-Small Cell Lung Cancer (NSCLC) : Drugs like gefitinib and osimertinib target specific mutations in EGFR, providing effective treatment options for patients with advanced disease .
- HER2-Positive Breast Cancer : Trastuzumab, a TKI targeting HER2, has become a cornerstone in treating this subtype of breast cancer, enhancing survival rates significantly .
Angiogenesis Inhibition
TKIs such as apatinib inhibit angiogenesis by blocking VEGFR signaling. This application has been explored in various solid tumors:
- Case Study : A 91-year-old patient with recurrent Merkel cell carcinoma showed a complete response to apatinib after four months of therapy, demonstrating its potential utility beyond traditional cancers .
Research Trends and Future Directions
- Resistance Mechanisms : Genetic mutations such as T790M can render first-generation TKIs ineffective. New generation TKIs are being developed to target these mutations specifically .
- Combination Therapies : Ongoing studies are investigating the efficacy of combining TKIs with other therapeutic modalities to enhance treatment outcomes and manage resistance .
Case Study 1: Apatinib in Merkel Cell Carcinoma
A patient with recurrent Merkel cell carcinoma received apatinib after surgery. The treatment led to a complete response within four months. Despite some manageable side effects like hypertension and proteinuria, the patient achieved 27 months of progression-free survival .
Case Study 2: Dasatinib in Chronic Myelogenous Leukemia
A patient who intermittently stopped dasatinib therapy maintained a deep molecular response for 18 months without treatment. Upon relapse, reinitiating dasatinib at a lower dose resulted in a major molecular response .
Mechanism of Action
Tyrosine kinase inhibitors work by blocking the enzyme activity of tyrosine kinases, preventing the phosphorylation of proteins involved in cell signaling pathways. This inhibition disrupts the signal transduction cascades that promote cancer cell growth and proliferation. Molecular targets include the epidermal growth factor receptor (EGFR), vascular endothelial growth factor receptor (VEGFR), and other receptor tyrosine kinases .
Comparison with Similar Compounds
Comparative Analysis of Tyrosine Kinase Inhibitors and Similar Compounds
Structural and Binding Mode Comparisons
TKIs exhibit structural diversity but share common features such as heterocyclic scaffolds (e.g., quinazolines, pyridines) that mimic ATP’s purine ring. For example:
- Erlotinib (an EGFR inhibitor) and structurally similar ligands from Trichoderma metabolites show >80% similarity in canonical SMILES structures, leading to comparable binding affinities in EGFR’s ATP-binding pocket .
- Vandetanib (ZD6474) , a multi-target TKI, shares a binding mode with sunitinib in VEGFR-2, as confirmed by molecular docking studies .
Key Finding : Structural analogs often occupy identical 3D positions in kinase binding sites, but subtle differences (e.g., substituent groups) can alter selectivity and resistance profiles .
Pharmacokinetic and Physicochemical Properties
TKIs face challenges in pharmacokinetic predictability due to interindividual metabolic variations and interactions with drug transporters like ABCB1 (Table 1):
Key Finding : Small physicochemical differences (e.g., logP, molecular weight) significantly influence bioavailability and resistance mechanisms .
Potency and Selectivity Profiles
TKIs vary in kinase inhibition potency, often quantified by IC50 values. However, cross-study comparisons are confounded by assay heterogeneity (Table 2):
Key Finding : Imatinib and its analog SKI696 exhibit overlapping selectivity for PDGFRα and c-Kit but differ in Abl inhibition .
Clinical Efficacy and Resistance Mechanisms
Resistance Profiles
- EGFR Mutations : The T790M mutation in EGFR confers resistance to gefitinib but is overcome by CL-387,785 , a covalent TKI with higher binding affinity .
Combination Therapy Synergy
- ZD-1839 (Gefitinib): Combined with paclitaxel or topotecan, it enhances apoptosis in GEO colon cancer xenografts, achieving tumor growth arrest .
Key Finding : Resistance mechanisms are compound-specific, necessitating tailored TKI combinations or next-generation inhibitors .
Biological Activity
Tyrosine kinase inhibitors (TKIs) represent a significant advancement in targeted cancer therapy, primarily due to their ability to interfere with the signaling pathways that regulate cell proliferation, survival, and migration. This article delves into the biological activity of TKIs, highlighting their mechanisms of action, therapeutic applications, resistance mechanisms, and associated case studies.
Overview of Tyrosine Kinases
Tyrosine kinases are enzymes that catalyze the transfer of phosphate groups from ATP to tyrosine residues on proteins, playing a crucial role in various cellular processes such as growth, differentiation, and metabolism. Dysregulation of these kinases is often implicated in oncogenesis, making them prime targets for therapeutic intervention.
TKIs function by competitively inhibiting the binding of ATP to the kinase domain, thereby blocking the phosphorylation of target proteins. This inhibition can lead to:
- Reduced cell proliferation : TKIs can halt the growth of cancer cells by disrupting key signaling pathways.
- Induction of apoptosis : By blocking survival signals, TKIs can promote programmed cell death in malignant cells.
- Inhibition of angiogenesis : Some TKIs target vascular endothelial growth factor receptors (VEGFRs), thereby impeding the formation of new blood vessels that tumors require for growth.
Types of Tyrosine Kinase Inhibitors
TKIs can be categorized into two main types:
- Small Molecule Inhibitors : These are typically orally administered drugs that penetrate cells easily.
- Monoclonal Antibodies : These larger molecules target specific receptors on the surface of cancer cells.
Type | Examples | Mechanism |
---|---|---|
Small Molecule Inhibitors | Imatinib, Erlotinib | ATP-competitive inhibition |
Monoclonal Antibodies | Trastuzumab, Cetuximab | Block receptor-ligand interactions |
Case Study: Chronic Myeloid Leukemia (CML)
A notable case involved a patient with CML who voluntarily discontinued TKI therapy after achieving deep molecular remission. Despite a relapse detected 18 months later, she maintained normal blood counts for an extended period without treatment. Transcriptome analysis revealed significant immune activity during this period, suggesting potential for immune surveillance in TKI discontinuation scenarios .
Research Findings
- Efficacy in Solid Tumors : TKIs such as erlotinib and osimertinib have shown substantial efficacy in treating non-small cell lung cancer (NSCLC), particularly in patients harboring specific mutations like T790M .
- Resistance Mechanisms : Resistance to TKIs can arise through various mechanisms including secondary mutations in the target kinase or activation of alternative signaling pathways. For instance, mutations like C797S have been identified as critical factors contributing to resistance .
- Adverse Effects : While TKIs are effective, they are also associated with a range of side effects including cardiovascular complications such as hypertension and atrial fibrillation, particularly noted with agents like dasatinib and ponatinib .
Q & A
Q. How can researchers design experiments to evaluate mutation-specific TKI sensitivity in non-small cell lung cancer (NSCLC)?
Methodological Approach :
- Step 1 : Perform genomic sequencing (e.g., EGFR exons 18–21) to identify mutations (e.g., exon 19 deletions, L858R) in NSCLC patient samples .
- Step 2 : Use isogenic cell lines engineered with specific mutations to compare TKI efficacy (e.g., gefitinib, erlotinib) via dose-response assays (IC50 calculations).
- Step 3 : Validate findings using patient-derived xenografts (PDXs) or 3D organoid models to assess in vivo sensitivity .
- Data Interpretation : Correlate mutation type with clinical response rates and progression-free survival in retrospective cohorts.
Table 1 : Example of EGFR Mutation-Specific TKI Sensitivity
Mutation Type | TKI Tested | IC50 (nM) | Clinical Response Rate |
---|---|---|---|
Exon 19 deletion | Gefitinib | 2.5 | 75% |
L858R (exon 21) | Erlotinib | 3.8 | 68% |
Wild-type EGFR | Gefitinib | >1000 | <10% |
Q. What advanced structural biology methods are used to design next-generation TKIs targeting resistance mutations?
Methodological Answer :
- Rational Drug Design :
- Use cryo-EM or X-ray crystallography to resolve drug-target complexes (e.g., RET or c-Src kinases) and identify cryptic/allosteric binding pockets .
- Perform molecular dynamics simulations to study conformational changes induced by resistance mutations (e.g., T790M in EGFR) .
- Screen virtual chemical libraries for compounds that stabilize inactive kinase conformations or exploit hydrophobic core regions .
- Validation : Test candidate inhibitors in biochemical assays (e.g., kinase activity profiling) and resistant cell lines .
Advanced Consideration : Prioritize compounds with polypharmacological profiles to counteract compensatory signaling pathways .
Q. How should researchers address contradictions between preclinical and clinical TKI efficacy data?
Analytical Framework :
- Step 1 : Evaluate preclinical models for limitations (e.g., lack of tumor microenvironment in cell lines vs. PDX models) .
- Step 2 : Use multi-omics integration (transcriptomics, proteomics) to identify biomarkers of discordance (e.g., stromal interactions, immune evasion).
- Step 3 : Apply Bayesian statistical models to reconcile heterogeneous clinical trial data, accounting for patient subpopulations and comorbidities .
- Case Study : Hepatocyte growth factor (HGF)-induced MET activation in nasopharyngeal carcinoma reduced TKI efficacy in vivo but not in vitro, highlighting the need for co-treatment strategies .
Q. What methodologies are recommended for systematic reviews of TKI safety and efficacy across diverse cancer types?
Guidelines :
- Follow PRISMA protocols for literature screening, data extraction, and bias assessment .
- Use tools like Covidence to manage multi-reviewer workflows and GRADE criteria for evidence quality grading .
- Focus Areas :
Q. How can researchers investigate mechanisms of acquired TKI resistance using longitudinal studies?
Experimental Design :
- Temporal Sampling : Collect serial biopsies or liquid biopsies (ctDNA) from patients pre-/post-TKI therapy to track clonal evolution .
- Functional Genomics : Perform CRISPR-Cas9 screens in TKI-resistant cells to identify upregulated bypass pathways (e.g., MET amplification, RAS mutations) .
- Single-Cell RNA Sequencing : Resolve intratumoral heterogeneity and rare resistant subpopulations .
Table 2 : Common Resistance Mechanisms and Counterstrategies
Q. What computational approaches optimize TKI combination therapy regimens?
Advanced Methodology :
- Network Pharmacology : Map kinase signaling cascades to identify synergistic targets (e.g., combining EGFR and MEK inhibitors) .
- Machine Learning : Train models on clinical trial datasets to predict optimal drug pairs and dosing schedules.
- In Vivo Validation : Use orthotopic tumor models to test combinations while monitoring toxicity via pharmacokinetic/pharmacodynamic (PK/PD) modeling .
Q. How do researchers validate allosteric TKI binding and its functional impact?
Stepwise Approach :
- Biophysical Assays : Surface plasmon resonance (SPR) or isothermal titration calorimetry (ITC) to measure binding kinetics .
- Cellular Assays : Monitor downstream signaling (e.g., phospho-ERK/STAT levels) via Western blot or Luminex multiplex assays .
- Structural Validation : Compare cryo-EM structures of allosteric vs. ATP-competitive inhibitors to confirm binding mode .
Properties
IUPAC Name |
1-N'-(4-fluorophenyl)-1-N-[4-[[2-(2-morpholin-4-ylethylcarbamoyl)-1H-pyrrolo[2,3-b]pyridin-4-yl]oxy]phenyl]cyclopropane-1,1-dicarboxamide | |
---|---|---|
Source | PubChem | |
URL | https://pubchem.ncbi.nlm.nih.gov | |
Description | Data deposited in or computed by PubChem | |
InChI |
InChI=1S/C31H31FN6O5/c32-20-1-3-21(4-2-20)35-29(40)31(10-11-31)30(41)36-22-5-7-23(8-6-22)43-26-9-12-33-27-24(26)19-25(37-27)28(39)34-13-14-38-15-17-42-18-16-38/h1-9,12,19H,10-11,13-18H2,(H,33,37)(H,34,39)(H,35,40)(H,36,41) | |
Source | PubChem | |
URL | https://pubchem.ncbi.nlm.nih.gov | |
Description | Data deposited in or computed by PubChem | |
InChI Key |
PKOVTRMHYNEBDU-UHFFFAOYSA-N | |
Source | PubChem | |
URL | https://pubchem.ncbi.nlm.nih.gov | |
Description | Data deposited in or computed by PubChem | |
Canonical SMILES |
C1CC1(C(=O)NC2=CC=C(C=C2)OC3=C4C=C(NC4=NC=C3)C(=O)NCCN5CCOCC5)C(=O)NC6=CC=C(C=C6)F | |
Source | PubChem | |
URL | https://pubchem.ncbi.nlm.nih.gov | |
Description | Data deposited in or computed by PubChem | |
Molecular Formula |
C31H31FN6O5 | |
Source | PubChem | |
URL | https://pubchem.ncbi.nlm.nih.gov | |
Description | Data deposited in or computed by PubChem | |
DSSTOX Substance ID |
DTXSID40648066 | |
Record name | N~1~-(4-Fluorophenyl)-N'~1~-{4-[(2-{[2-(morpholin-4-yl)ethyl]carbamoyl}-1H-pyrrolo[2,3-b]pyridin-4-yl)oxy]phenyl}cyclopropane-1,1-dicarboxamide | |
Source | EPA DSSTox | |
URL | https://comptox.epa.gov/dashboard/DTXSID40648066 | |
Description | DSSTox provides a high quality public chemistry resource for supporting improved predictive toxicology. | |
Molecular Weight |
586.6 g/mol | |
Source | PubChem | |
URL | https://pubchem.ncbi.nlm.nih.gov | |
Description | Data deposited in or computed by PubChem | |
CAS No. |
1021950-26-4 | |
Record name | N~1~-(4-Fluorophenyl)-N'~1~-{4-[(2-{[2-(morpholin-4-yl)ethyl]carbamoyl}-1H-pyrrolo[2,3-b]pyridin-4-yl)oxy]phenyl}cyclopropane-1,1-dicarboxamide | |
Source | EPA DSSTox | |
URL | https://comptox.epa.gov/dashboard/DTXSID40648066 | |
Description | DSSTox provides a high quality public chemistry resource for supporting improved predictive toxicology. | |
Retrosynthesis Analysis
AI-Powered Synthesis Planning: Our tool employs the Template_relevance Pistachio, Template_relevance Bkms_metabolic, Template_relevance Pistachio_ringbreaker, Template_relevance Reaxys, Template_relevance Reaxys_biocatalysis model, leveraging a vast database of chemical reactions to predict feasible synthetic routes.
One-Step Synthesis Focus: Specifically designed for one-step synthesis, it provides concise and direct routes for your target compounds, streamlining the synthesis process.
Accurate Predictions: Utilizing the extensive PISTACHIO, BKMS_METABOLIC, PISTACHIO_RINGBREAKER, REAXYS, REAXYS_BIOCATALYSIS database, our tool offers high-accuracy predictions, reflecting the latest in chemical research and data.
Strategy Settings
Precursor scoring | Relevance Heuristic |
---|---|
Min. plausibility | 0.01 |
Model | Template_relevance |
Template Set | Pistachio/Bkms_metabolic/Pistachio_ringbreaker/Reaxys/Reaxys_biocatalysis |
Top-N result to add to graph | 6 |
Feasible Synthetic Routes
Disclaimer and Information on In-Vitro Research Products
Please be aware that all articles and product information presented on BenchChem are intended solely for informational purposes. The products available for purchase on BenchChem are specifically designed for in-vitro studies, which are conducted outside of living organisms. In-vitro studies, derived from the Latin term "in glass," involve experiments performed in controlled laboratory settings using cells or tissues. It is important to note that these products are not categorized as medicines or drugs, and they have not received approval from the FDA for the prevention, treatment, or cure of any medical condition, ailment, or disease. We must emphasize that any form of bodily introduction of these products into humans or animals is strictly prohibited by law. It is essential to adhere to these guidelines to ensure compliance with legal and ethical standards in research and experimentation.