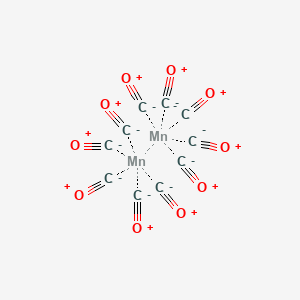
Manganese carbonyl
Overview
Description
Manganese carbonyl is a reagent used in organometallic reactions to prepare polynuclear selenium this compound cluster complexes .
Synthesis Analysis
This compound compounds have been synthesized for various purposes. For instance, new manganese metal carbonyl compounds that contain sulfur and selenium ligands have been synthesized and evaluated for their potential use as photoCORMs .
Molecular Structure Analysis
This compound has the chemical formula Mn2(CO)10 . The electronic structure of Mn2(CO)10 was reported in 2017 using the BP86D functional with TZP basis set .
Chemical Reactions Analysis
This compound complexes have been used in various chemical reactions. For example, they have been used as catalysts for the hydrogenation of quinolines and imines . They have also been studied for their photochemistry within a permanently porous metal–organic framework .
Physical And Chemical Properties Analysis
This compound has a molecular weight of 389.98 g/mol . More detailed physical and chemical properties would require specific experimental measurements or computational simulations.
Scientific Research Applications
Luminescence and Photoreactivity
Manganese carbonyl complexes exhibit unique properties related to luminescence and photoreactivity. These complexes are studied for their potential applications in photochemistry and photophysics. The research in this area focuses on understanding the chemical structure and behavior of this compound complexes under different conditions, particularly how they react to light and other forms of electromagnetic radiation.
Catalytic Applications
This compound compounds play a crucial role in catalysis. They are involved in various catalytic processes such as:
- Hydrogenation of nitriles and ketones.
- Catalyst design and E-H bond activation.
- Additive-free hydrogenation of nitriles.
- Hydrogenation of α,β-unsaturated ketones.
- Reduction of ketones.
- Lewis acid-assisted hydrogenation of carbon dioxide to formate.
- Hydrogenation of mono- and disubstituted alkenes.
- Semihydrogenation of alkynes.
- Hydroboration of alkenes and trans-1,2-diboration of terminal alkynes.
- Dehydrogenative silylation of alkenes yielding vinyl- and allyl silanes.
- Dimerization and cross-coupling of terminal alkynes
.
Mechanism of Action
Target of Action
Manganese carbonyl, also known as Manganese(0) carbonyl or Mn2(CO)10, primarily targets cancer cells and multidrug-resistant Gram-negative bacteria . In cancer cells, it shows a selectivity towards ovarian carcinoma cell lines . In the case of bacteria, it has shown activity against multidrug-resistant clinical isolates of Acinetobacter baumannii and Pseudomonas aeruginosa .
Mode of Action
This compound complexes interact with their targets primarily through the generation of reactive oxygen species (ROS) and the release of carbon monoxide (CO) . The antiproliferative activity of these complexes has been observed to be dose-dependent, with a higher effect in complex 1 and 2 .
Biochemical Pathways
The this compound complexes are involved in several biochemical pathways. They exhibit behaviors for controlled CO-release by both thermal and photosynthetic pathways . In cancer cells, exposure to these complexes leads to an increase in ROS, which activates cell death mechanisms, namely via intrinsic apoptosis for complex 2 and autophagy and extrinsic apoptosis for complex 1 .
Pharmacokinetics
The pharmacokinetics of this compound complexes are influenced by their nanomaterial form. The uniform and stable spherical nanoparticles enhance drug absorption and ensure mitochondrial targeted delivery . This increases intracellular ROS and highly toxic hydroxyl radicals (˙OH), thereby effectively inhibiting the proliferation of both chemosensitive and chemoresistant MCF-7 breast cancer cells .
Result of Action
The result of the action of this compound complexes is the inhibition of cell proliferation. In cancer cells, these complexes are able to potentiate cell migration and neovascularization, indicating that their application might be directed for initial tumor stages to avoid tumor invasion and metastasis .
Action Environment
The action of this compound complexes is influenced by environmental factors such as the presence of weak Brønsted acids . These complexes only show CO2 reduction activity with the addition of weak Brønsted acids . The selectivity of these catalysts in the presence of Brønsted acids is due to a proton-assisted CO2 binding mechanism .
Safety and Hazards
Biochemical Analysis
Molecular Mechanism
It’s known that Manganese Carbonyl can act as a reducing agent, which could potentially influence redox reactions at the molecular level
Temporal Effects in Laboratory Settings
This compound is not very stable in air, to heat, or UV light . It decomposes on heating to 250°C
properties
IUPAC Name |
carbon monoxide;manganese | |
---|---|---|
Source | PubChem | |
URL | https://pubchem.ncbi.nlm.nih.gov | |
Description | Data deposited in or computed by PubChem | |
InChI |
InChI=1S/10CO.2Mn/c10*1-2;; | |
Source | PubChem | |
URL | https://pubchem.ncbi.nlm.nih.gov | |
Description | Data deposited in or computed by PubChem | |
InChI Key |
QFEOTYVTTQCYAZ-UHFFFAOYSA-N | |
Source | PubChem | |
URL | https://pubchem.ncbi.nlm.nih.gov | |
Description | Data deposited in or computed by PubChem | |
Canonical SMILES |
[C-]#[O+].[C-]#[O+].[C-]#[O+].[C-]#[O+].[C-]#[O+].[C-]#[O+].[C-]#[O+].[C-]#[O+].[C-]#[O+].[C-]#[O+].[Mn].[Mn] | |
Source | PubChem | |
URL | https://pubchem.ncbi.nlm.nih.gov | |
Description | Data deposited in or computed by PubChem | |
Molecular Formula |
C10Mn2O10 | |
Source | PubChem | |
URL | https://pubchem.ncbi.nlm.nih.gov | |
Description | Data deposited in or computed by PubChem | |
Molecular Weight |
389.98 g/mol | |
Source | PubChem | |
URL | https://pubchem.ncbi.nlm.nih.gov | |
Description | Data deposited in or computed by PubChem | |
CAS RN |
10170-69-1 | |
Record name | Dimanganese decacarbonyl | |
Source | CAS Common Chemistry | |
URL | https://commonchemistry.cas.org/detail?cas_rn=10170-69-1 | |
Description | CAS Common Chemistry is an open community resource for accessing chemical information. Nearly 500,000 chemical substances from CAS REGISTRY cover areas of community interest, including common and frequently regulated chemicals, and those relevant to high school and undergraduate chemistry classes. This chemical information, curated by our expert scientists, is provided in alignment with our mission as a division of the American Chemical Society. | |
Explanation | The data from CAS Common Chemistry is provided under a CC-BY-NC 4.0 license, unless otherwise stated. | |
Record name | Manganese carbonyl | |
Source | DTP/NCI | |
URL | https://dtp.cancer.gov/dtpstandard/servlet/dwindex?searchtype=NSC&outputformat=html&searchlist=203018 | |
Description | The NCI Development Therapeutics Program (DTP) provides services and resources to the academic and private-sector research communities worldwide to facilitate the discovery and development of new cancer therapeutic agents. | |
Explanation | Unless otherwise indicated, all text within NCI products is free of copyright and may be reused without our permission. Credit the National Cancer Institute as the source. | |
Record name | Manganese carbonyl | |
Source | DTP/NCI | |
URL | https://dtp.cancer.gov/dtpstandard/servlet/dwindex?searchtype=NSC&outputformat=html&searchlist=22319 | |
Description | The NCI Development Therapeutics Program (DTP) provides services and resources to the academic and private-sector research communities worldwide to facilitate the discovery and development of new cancer therapeutic agents. | |
Explanation | Unless otherwise indicated, all text within NCI products is free of copyright and may be reused without our permission. Credit the National Cancer Institute as the source. | |
Record name | Decacarbonyldimanganese | |
Source | European Chemicals Agency (ECHA) | |
URL | https://echa.europa.eu/substance-information/-/substanceinfo/100.030.392 | |
Description | The European Chemicals Agency (ECHA) is an agency of the European Union which is the driving force among regulatory authorities in implementing the EU's groundbreaking chemicals legislation for the benefit of human health and the environment as well as for innovation and competitiveness. | |
Explanation | Use of the information, documents and data from the ECHA website is subject to the terms and conditions of this Legal Notice, and subject to other binding limitations provided for under applicable law, the information, documents and data made available on the ECHA website may be reproduced, distributed and/or used, totally or in part, for non-commercial purposes provided that ECHA is acknowledged as the source: "Source: European Chemicals Agency, http://echa.europa.eu/". Such acknowledgement must be included in each copy of the material. ECHA permits and encourages organisations and individuals to create links to the ECHA website under the following cumulative conditions: Links can only be made to webpages that provide a link to the Legal Notice page. | |
Q & A
Q1: What is the molecular formula and weight of Mn2(CO)10?
A1: Mn2(CO)10 has a molecular formula of C10Mn2O10 and a molecular weight of 349.94 g/mol.
Q2: What spectroscopic techniques are commonly used to characterize Mn2(CO)10?
A2: Mn2(CO)10 is frequently studied using various spectroscopic methods, including:
- Infrared (IR) spectroscopy: IR spectroscopy is particularly useful for analyzing the carbonyl (CO) stretching vibrations in Mn2(CO)10. [, , ] This helps in identifying the compound and studying its interactions with other molecules.
- Nuclear Magnetic Resonance (NMR) spectroscopy: While less common due to Mn2(CO)10's structure, 55Mn NMR at high magnetic fields can provide insights into the chemical environment of manganese atoms within the complex. []
- Extended X-ray Absorption Fine Structure (EXAFS) spectroscopy: EXAFS is helpful in determining bond lengths and coordination environments around the manganese atoms in Mn2(CO)10 and its derivatives. [, ]
Q3: How does the stability of Mn2(CO)10 vary in different solvents?
A: The stability of Mn2(CO)10 is solvent-dependent. It generally exhibits better stability in non-polar hydrocarbon solvents compared to polar solvents. [, ]
Q4: What happens to Mn2(CO)10 upon exposure to light?
A: Mn2(CO)10 is photosensitive and readily undergoes photolysis upon exposure to UV or visible light, leading to the formation of Mn(CO)5 and other species. [, , , , ] This property has been exploited for applications in photochemistry and photocatalysis.
Q5: Can Mn2(CO)10 act as a catalyst?
A: While Mn2(CO)10 itself might have limited catalytic activity, it can act as a precatalyst. Upon activation, often through photolysis or reaction with other reagents, it generates catalytically active species. [, , , ]
Q6: What types of reactions can be catalyzed by Mn2(CO)10-derived species?
A6: Mn2(CO)10-derived species have been explored as catalysts for various reactions, including:
- Hydrosilation of ketones: Mn2(CO)10 and its derivatives have shown promising activity in catalyzing the hydrosilation of ketones, demonstrating even higher reactivity than traditional catalysts like Rh(PPh3)3Cl in certain cases. [, ]
- Polymerization reactions: Mn2(CO)10, in conjunction with specific co-reagents, can initiate and mediate radical polymerization processes, enabling the synthesis of polymers with controlled architectures like block copolymers. [, , , ]
- CO release: Mn2(CO)10 is known to release CO upon photoactivation, making it a potential candidate for developing photo-activated CO-releasing molecules (photoCORMs) with possible therapeutic applications. [, , ]
Q7: How has computational chemistry been used to study Mn2(CO)10?
A7: Density functional theory (DFT) calculations have been extensively employed to:
- Investigate the structures and stabilities of Mn2(CO)10 and its derivatives: DFT helps predict the geometries of different isomers, bond strengths, and relative energies of various species. [, , ]
- Elucidate reaction mechanisms: Computational studies can provide insights into the intermediates and transition states involved in reactions catalyzed by Mn2(CO)10-derived species. []
Q8: How does modification of the CO ligands in Mn2(CO)10 affect its properties?
A: Replacing CO ligands with other groups like phosphines, halides, or thiolates can significantly alter Mn2(CO)10's reactivity, stability, and catalytic activity. [, , , ] These modifications can tune the electronic and steric properties around the manganese center, influencing its interactions with other molecules and its performance in various applications.
Q9: Are there any alternative compounds with similar properties to Mn2(CO)10 for specific applications?
A9: Yes, depending on the application, several alternatives to Mn2(CO)10 exist:
- PhotoCORMs: Other transition metal carbonyl complexes, such as those based on iron or ruthenium, are being explored as potential photoCORMs. [, ]
- Catalysts: Depending on the desired transformation, numerous other transition metal complexes, including those based on rhodium, palladium, or copper, can offer comparable or even superior catalytic activities. []
- Polymerization initiators: Various other initiators, such as azo compounds or peroxides, are widely used for radical polymerization reactions. []
Disclaimer and Information on In-Vitro Research Products
Please be aware that all articles and product information presented on BenchChem are intended solely for informational purposes. The products available for purchase on BenchChem are specifically designed for in-vitro studies, which are conducted outside of living organisms. In-vitro studies, derived from the Latin term "in glass," involve experiments performed in controlled laboratory settings using cells or tissues. It is important to note that these products are not categorized as medicines or drugs, and they have not received approval from the FDA for the prevention, treatment, or cure of any medical condition, ailment, or disease. We must emphasize that any form of bodily introduction of these products into humans or animals is strictly prohibited by law. It is essential to adhere to these guidelines to ensure compliance with legal and ethical standards in research and experimentation.