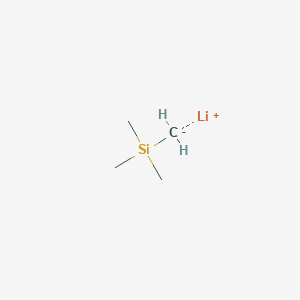
(Trimethylsilyl)methyllithium
Overview
Description
(Trimethylsilyl)methyllithium (LiCH2SiMe3) is a highly reactive organolithium reagent widely employed in synthetic chemistry. Its structure in the solid state is hexameric, as confirmed by X-ray crystallography, with Li–C bond lengths ranging from 2.15 Å to 2.29 Å in the [Li6C6] core . The compound exhibits spatial flexibility due to the bulky trimethylsilyl group, enabling its use in forming otherwise inaccessible metal complexes . Key applications include:
Preparation Methods
Classical Alkali Metal Halide Exchange
The most widely employed strategy for synthesizing LiCH₂SiMe₃ involves alkali metal-halogen exchange, leveraging the reactivity of organolithium precursors with (trimethylsilyl)methyl halides.
Butyllithium-Mediated Synthesis
The reaction of (trimethylsilyl)methyl chloride (Me₃SiCH₂Cl) with butyllithium (BuLi) in ethereal solvents constitutes the benchmark method:
3\text{SiCH}2\text{Cl} + \text{BuLi} \rightarrow \text{LiCH}2\text{SiMe}3 + \text{BuCl}
Conducted under inert atmospheres at low temperatures (−78°C to 0°C), this method typically employs tetrahydrofuran (THF) or hexanes as solvents . The exothermic reaction necessitates controlled addition to mitigate side reactions, such as Wurtz coupling. While yields are often high (80–90%), the procedure demands rigorous exclusion of moisture and oxygen to prevent reagent decomposition .
Lithium Dendrite-Mediated Synthesis
A recent advancement utilizes highly reactive lithium metal dendrites to enhance reaction efficiency. Gonzalez et al. demonstrated that treating Me₃SiCH₂Cl with lithium dendrites (prepared via liquid ammonia etching) in THF/hexanes at 0°C affords LiCH₂SiMe₃ in 86–94% yield . The dendritic lithium’s increased surface area accelerates the metal-halogen exchange, reducing reaction times and improving reproducibility . This method is particularly advantageous for sterically hindered substrates, as evidenced by successful applications in tertiary alkyl lithium syntheses .
Grignard Reagent Transmetalation
An alternative route involves transmetalation of (trimethylsilyl)methylmagnesium chloride (Me₃SiCH₂MgCl) with lithium salts:
3\text{SiCH}2\text{MgCl} + \text{LiX} \rightarrow \text{LiCH}2\text{SiMe}3 + \text{MgXCl}
While less common due to the additional step of preparing the Grignard reagent, this method offers milder conditions (0°C to room temperature) and compatibility with ether solvents . However, yields are generally lower (70–80%) compared to direct lithium-halogen exchange, and the method is less favored for large-scale synthesis .
Comparative Analysis of Synthetic Methods
The choice of method hinges on substrate sensitivity, scalability, and desired purity. Below is a comparative overview:
Chemical Reactions Analysis
Nucleophilic Substitution Reactions
LiCH₂SiMe₃ acts as a strong nucleophile, forming carbon-carbon bonds with electrophiles:
-
Aldehydes/Ketones : Reacts to form alcohols after hydrolysis (Peterson olefination). For example, with benzaldehyde:
Electron-rich benzaldehydes react faster than electron-deficient ones due to enhanced electrophilicity .
-
Epoxides : Undergoes ring-opening at −5°C in THF to form secondary alcohols (e.g., TsiCH₂CH₂OH) . Steric hindrance in bulky epoxides (e.g., cyclohexene oxide) leads to proton abstraction instead .
Table 1: Reaction Conditions and Products with Carbonyl Compounds
Substrate | Product | Conditions | Yield (%) | Source |
---|---|---|---|---|
Benzaldehyde | Benzyl alcohol | THF, −78°C, 1 h | 85 | |
Acetophenone | 1-Phenylethanol | THF, 0°C, 2 h | 78 | |
Ethylene oxide | TsiCH₂CH₂OH | THF, 0°C, 60 min | 92 |
Organometallic Complex Formation
LiCH₂SiMe₃ facilitates the synthesis of sterically hindered organometallic complexes:
-
Reacts with metal chlorides (e.g., TiCl₄, FeCl₃) via salt metathesis to form [(Me₃Si)CH₂]ₙM complexes .
-
Monomeric [Li(CH₂SiMe₃)(Me₆Tren)] exhibits enhanced Li–C bond reactivity, enabling CO insertion and toluene C–H activation .
Key Insight : Monomeric forms show 10× faster C–H activation rates compared to hexameric aggregates .
Polymer Modification
LiCH₂SiMe₃ modifies polymers via epoxy ring-opening:
-
Reacts with poly(glycidyl methacrylate-co-2-ethylhexyl methacrylate) (GMA-co-EHMA) at −5°C, attaching tris(trimethylsilyl)methyl groups to the polymer backbone .
-
Evidence : FT-IR shows disappearance of epoxide peaks (907 cm⁻¹) and emergence of C–Si bands (900, 1250 cm⁻¹) .
Halogenation and Esterification
TsiCH₂CH₂OH (from ethylene oxide reaction) undergoes further derivatization:
-
Halogenation : Treatment with SOCl₂ yields TsiCH₂CH₂Cl (92% yield) .
-
Esterification : Reacts with acyl chlorides (e.g., AcCl) to form TsiCH₂CH₂OAc .
Silicon Rearrangement and Fragmentation
LiCH₂SiMe₃ participates in oligosilane rearrangements:
-
With AlCl₃, n-octadecamethyloctasilane fragments into 2,2,4-tris(trimethylsilyl)nonamethylpentasilane (4 ) and cyclopentasilane derivatives .
-
Mechanism : 1,5-silyl shifts occur, releasing trimethylsilylium ions .
Table 2: Reactivity with Silicon Substrates
Substrate | Major Product | Conditions | Source |
---|---|---|---|
n-Octadecamethyloctasilane | 4 (81%), 5 (19%) | AlCl₃, CH₂Cl₂, RT | |
2,5-Bis(TMS)dodecamethylhexasilane | Cyclopentasilane (7 ) | Al(Fe)Cl₃, 24 h |
Stability and Solvent Effects
-
Stability : Hexameric [LiCH₂SiMe₃]₆ is stable in THF, but monomeric forms decompose via C–H/N–C bond cleavage .
-
Solvent : Reactions in THF/Me₂O show rapid kinetics (k ≥ 2 s⁻¹ at −130°C) . HMPA additives decelerate reactivity by stabilizing Li⁺ .
Comparative Reactivity
Table 3: Aggregation State vs. Reactivity
Form | Reactivity Highlights | Source |
---|---|---|
Hexamer ([LiR]₆) | Stable but slower in C–H activation | |
Monomer ([LiR]) | Enhanced CO insertion and toluene C–H activation |
Limitations
Scientific Research Applications
1.1. Carbon-Carbon Bond Formation
TMSLi is widely used for forming carbon-carbon bonds through various coupling reactions. One notable application is in the C(sp²) cross-coupling reactions , where TMSLi reacts with various electrophiles to generate structurally diverse products. For instance, the use of palladium catalysts facilitates the coupling of TMSLi with aryl halides, allowing for the synthesis of benzyl and related compounds .
1.2. Cycloaddition Reactions
TMSLi has been utilized in formal [3+2] cycloadditions with electron-deficient olefins, demonstrating its ability to form complex cyclic structures. This methodology has been applied to synthesize α-tert-alkyamino carboxylic esters, which are precursors for biologically active compounds . The protonolytic ring-opening of intermediates generated from these reactions leads to valuable synthetic pathways for natural products.
Reaction Type | Substrates | Products |
---|---|---|
C(sp²) Cross-Coupling | Aryl Halides + TMSLi | Benzyl Compounds |
[3+2] Cycloaddition | Electron-deficient Olefins + TMSLi | α-Tert-Alkyamino Carboxylic Esters |
1.3. Tandem Reactions
In tandem reaction processes, TMSLi facilitates multiple bond formations and fragmentations in a single reaction vessel. This capability has been exploited to synthesize new heterocycles such as pyrazoles and diazepine derivatives . Such methodologies enhance synthetic efficiency by reducing the number of steps required in traditional synthesis.
2.1. Thin Film Deposition
TMSLi is also employed in material science, particularly in thin film deposition techniques used for creating semiconductor materials and coatings. Its reactivity allows it to function as a precursor for silicon-containing thin films, which are crucial in the electronics industry .
2.2. Polymer Chemistry
In polymer chemistry, TMSLi acts as a catalyst or initiator for polymerization reactions, contributing to the development of novel polymeric materials with tailored properties. Its ability to form stable complexes with various ligands enhances its utility in synthesizing functionalized polymers .
Organometallic Chemistry
TMSLi serves as an important precursor for synthesizing other organometallic compounds. Its stability and reactivity allow for the formation of various metal complexes that are valuable in catalysis and materials synthesis . The structural characteristics of TMSLi enable it to coordinate effectively with transition metals, facilitating diverse catalytic processes.
Case Study 1: Synthesis of Natural Products
A study demonstrated the application of TMSLi in synthesizing the amathaspiramide family of natural products through a series of cycloadditions and fragmentations, showcasing its effectiveness in complex molecule construction .
Case Study 2: Polymerization Initiation
Research highlighted TMSLi's role as an initiator in living anionic polymerization processes, leading to well-defined block copolymers with precise molecular weights and architectures .
Mechanism of Action
The mechanism of action of (Trimethylsilyl)methyllithium involves the formation of highly reactive intermediates that can participate in various chemical reactions. The lithium-carbon bond in the compound is highly polar, making the carbon atom nucleophilic. This allows it to attack electrophilic centers in other molecules, leading to the formation of new bonds .
Comparison with Similar Compounds
Comparative Analysis with Similar Organometallic Compounds
Structural and Bonding Differences
Key Observations :
- The hexameric structure of this compound contrasts with the monomeric bisthis compound, which adopts shorter Li–C bonds when coordinated with ligands like TMEDA .
- Methyllithium’s reactivity is often compromised by lithium halide impurities, whereas this compound avoids this issue due to its distinct synthesis .
Solubility and Reactivity
Key Observations :
- The trimethylsilyl group enhances solubility in nonpolar solvents, unlike methyllithium, which requires ethers .
- Sodium analogs (e.g., NaCH(SiMe3)2) exhibit unique solubility but lack the bifunctional utility of this compound .
Key Observations :
Biological Activity
(Trimethylsilyl)methyllithium, commonly referred to as LiCH₂Si(CH₃)₃, is an organolithium compound that exhibits significant reactivity in organic synthesis. Its biological activity has garnered interest due to its potential applications in medicinal chemistry and materials science. This article explores the biological activity of this compound, focusing on its reactivity patterns, synthesis, and implications for biological systems.
This compound is synthesized through the reaction of (trimethylsilyl)methyl chloride with butyllithium:
This compound crystallizes as a hexagonal prismatic hexamer and can form various adducts with different ligands, enhancing its stability and reactivity in solution .
Reactivity with Biological Molecules
Recent studies have highlighted the reactivity of this compound with biological substrates. For instance, it has been shown to react with aldehydes and ketones, facilitating the formation of carbon-carbon bonds, which is crucial in synthesizing complex organic molecules .
Table 1: Reactivity of this compound with Biological Substrates
Substrate | Reaction Type | Product |
---|---|---|
Aldehydes | Nucleophilic addition | Alcohol derivatives |
Ketones | Nucleophilic addition | Alcohol derivatives |
Epoxides | Ring-opening reaction | Alcohols |
Case Studies
- Reactivity with Epoxides : A study demonstrated that tristhis compound reacts with epoxides to form carbon-carbon bonds, highlighting its utility in constructing complex molecules relevant to pharmaceutical applications .
- NMR Studies : Research utilizing NMR spectroscopy has revealed insights into the structure and dynamics of this compound in solution. The steric bulk of the trimethylsilyl group influences its reactivity and interaction with other molecules, making it a valuable tool in synthetic organic chemistry .
Implications for Medicinal Chemistry
The ability of this compound to facilitate carbon-carbon bond formation positions it as a promising reagent in the development of new pharmaceuticals. Its unique properties allow for selective reactions that can lead to the synthesis of biologically active compounds.
Potential Applications
- Synthesis of Anticancer Agents : The compound's reactivity could be harnessed to synthesize novel anticancer agents by constructing complex molecular frameworks.
- Drug Development : The ability to modify existing drug structures through selective reactions opens avenues for improving efficacy and reducing side effects.
Q & A
Basic Research Questions
Q. What are the critical factors affecting the stability of (trimethylsilyl)methyllithium in solution, and how can its reactivity be preserved during storage?
this compound is typically stored as a 0.55 M solution in hexanes under inert conditions (argon/nitrogen) to prevent decomposition. Hexanes provide a low-polarity medium that stabilizes the organolithium species by minimizing solvent-Li+ interactions. Prolonged storage at low temperatures (e.g., −20°C) is recommended, as elevated temperatures accelerate decomposition via β-hydride elimination or hydrolysis. Analytical techniques like <sup>1</sup>H NMR or titration with diphenylacetic acid can monitor its concentration over time .
Q. How is this compound synthesized, and what purity benchmarks are essential for its use in reactions?
The reagent is prepared via transmetallation between (trimethylsilyl)methyl chloride and lithium metal in anhydrous hexanes. Critical purity benchmarks include:
- Residual chloride content (<0.1% by AgNO3 titration).
- Absence of LiCl precipitates (filtered via cannula transfer under inert atmosphere).
- Consistency in solution molarity (validated by quench-and-weigh methods). Impurities like LiCl or residual solvents (e.g., THF) can alter reactivity, necessitating rigorous characterization before use .
Q. What are the primary applications of this compound in foundational organometallic chemistry?
It is widely used as a strong nucleophile for silyl-methyl group transfer. Key reactions include:
- Silylative alkylation : Reacts with aldehydes/ketones to form β-silyl alcohols.
- Cross-coupling : Participates in Kumada or Negishi couplings with transition metal catalysts.
- Polymerization initiator : Generates silyl-terminated polymers in anionic polymerization. These applications require strict control of stoichiometry and temperature (−78°C to 0°C) to avoid side reactions .
Advanced Research Questions
Q. How does solvent polarity influence the reactivity of this compound in nucleophilic additions, and what mechanistic insights explain this behavior?
Solvent polarity modulates the ion-pairing equilibrium between the contact ion pair (CIP) and solvent-separated ion pair (SSIP). In low-polarity solvents (hexanes), the CIP dominates, reducing nucleophilicity. In polar solvents (THF), SSIP formation enhances reactivity due to increased Li+ solvation. Kinetic studies using rapid-injection NMR (RINMR) reveal that SSIPs exhibit faster reaction rates with electrophiles like aldehydes, but competing decomposition pathways (e.g., proton abstraction from THF) limit practical utility .
Q. What strategies resolve contradictions in reported yields for silyl-methylation reactions using this compound?
Discrepancies often arise from:
- Traces of moisture : Even ppm-level H2O hydrolyzes the reagent, reducing effective concentration.
- Substrate steric effects : Bulky electrophiles (e.g., aryl ketones) slow nucleophilic attack, favoring elimination side products.
- Temperature gradients : Localized heating during addition destabilizes the reagent. Solutions include:
- Pre-drying substrates with molecular sieves.
- Using cryogenic reactors for precise temperature control (−78°C ± 2°C).
- Employing excess reagent (1.2–1.5 equiv) to offset decomposition .
Q. How can kinetic isotope effects (KIEs) elucidate the mechanism of this compound-mediated C–Si bond formation?
Deuterium labeling at the methyl group (CD3)SiCH2Li enables measurement of primary KIEs. A KIE >1 indicates a transition state with significant C–Li bond cleavage (concerted mechanism), while KIE ≈1 suggests a pre-equilibrium (stepwise mechanism). RINMR studies on aldehyde additions show KIE values of 2.1–2.5, supporting a concerted pathway where Li+ coordination to the carbonyl oxygen precedes nucleophilic attack .
Q. How should researchers report conflicting data on this compound’s stability in published studies?
- Transparent documentation : Disclose solvent batch numbers, drying protocols, and storage durations.
- Side-reaction accounting : Quantify byproducts (e.g., trimethylsilane) via GC-MS or <sup>1</sup>H NMR integration.
- Meta-analysis : Compare datasets across studies using standardized metrics (e.g., half-life at −20°C). This approach clarifies whether discrepancies stem from methodological differences or intrinsic reagent variability .
Properties
IUPAC Name |
lithium;methanidyl(trimethyl)silane | |
---|---|---|
Source | PubChem | |
URL | https://pubchem.ncbi.nlm.nih.gov | |
Description | Data deposited in or computed by PubChem | |
InChI |
InChI=1S/C4H11Si.Li/c1-5(2,3)4;/h1H2,2-4H3;/q-1;+1 | |
Source | PubChem | |
URL | https://pubchem.ncbi.nlm.nih.gov | |
Description | Data deposited in or computed by PubChem | |
InChI Key |
KVWLUDFGXDFFON-UHFFFAOYSA-N | |
Source | PubChem | |
URL | https://pubchem.ncbi.nlm.nih.gov | |
Description | Data deposited in or computed by PubChem | |
Canonical SMILES |
[Li+].C[Si](C)(C)[CH2-] | |
Source | PubChem | |
URL | https://pubchem.ncbi.nlm.nih.gov | |
Description | Data deposited in or computed by PubChem | |
Molecular Formula |
C4H11LiSi | |
Source | PubChem | |
URL | https://pubchem.ncbi.nlm.nih.gov | |
Description | Data deposited in or computed by PubChem | |
DSSTOX Substance ID |
DTXSID90392901 | |
Record name | (Trimethylsilyl)methyllithium | |
Source | EPA DSSTox | |
URL | https://comptox.epa.gov/dashboard/DTXSID90392901 | |
Description | DSSTox provides a high quality public chemistry resource for supporting improved predictive toxicology. | |
Molecular Weight |
94.2 g/mol | |
Source | PubChem | |
URL | https://pubchem.ncbi.nlm.nih.gov | |
Description | Data deposited in or computed by PubChem | |
CAS No. |
1822-00-0 | |
Record name | (Trimethylsilyl)methyllithium | |
Source | EPA DSSTox | |
URL | https://comptox.epa.gov/dashboard/DTXSID90392901 | |
Description | DSSTox provides a high quality public chemistry resource for supporting improved predictive toxicology. | |
Record name | lithium;methanidyl(trimethyl)silane | |
Source | European Chemicals Agency (ECHA) | |
URL | https://echa.europa.eu/information-on-chemicals | |
Description | The European Chemicals Agency (ECHA) is an agency of the European Union which is the driving force among regulatory authorities in implementing the EU's groundbreaking chemicals legislation for the benefit of human health and the environment as well as for innovation and competitiveness. | |
Explanation | Use of the information, documents and data from the ECHA website is subject to the terms and conditions of this Legal Notice, and subject to other binding limitations provided for under applicable law, the information, documents and data made available on the ECHA website may be reproduced, distributed and/or used, totally or in part, for non-commercial purposes provided that ECHA is acknowledged as the source: "Source: European Chemicals Agency, http://echa.europa.eu/". Such acknowledgement must be included in each copy of the material. ECHA permits and encourages organisations and individuals to create links to the ECHA website under the following cumulative conditions: Links can only be made to webpages that provide a link to the Legal Notice page. | |
Retrosynthesis Analysis
AI-Powered Synthesis Planning: Our tool employs the Template_relevance Pistachio, Template_relevance Bkms_metabolic, Template_relevance Pistachio_ringbreaker, Template_relevance Reaxys, Template_relevance Reaxys_biocatalysis model, leveraging a vast database of chemical reactions to predict feasible synthetic routes.
One-Step Synthesis Focus: Specifically designed for one-step synthesis, it provides concise and direct routes for your target compounds, streamlining the synthesis process.
Accurate Predictions: Utilizing the extensive PISTACHIO, BKMS_METABOLIC, PISTACHIO_RINGBREAKER, REAXYS, REAXYS_BIOCATALYSIS database, our tool offers high-accuracy predictions, reflecting the latest in chemical research and data.
Strategy Settings
Precursor scoring | Relevance Heuristic |
---|---|
Min. plausibility | 0.01 |
Model | Template_relevance |
Template Set | Pistachio/Bkms_metabolic/Pistachio_ringbreaker/Reaxys/Reaxys_biocatalysis |
Top-N result to add to graph | 6 |
Feasible Synthetic Routes
Disclaimer and Information on In-Vitro Research Products
Please be aware that all articles and product information presented on BenchChem are intended solely for informational purposes. The products available for purchase on BenchChem are specifically designed for in-vitro studies, which are conducted outside of living organisms. In-vitro studies, derived from the Latin term "in glass," involve experiments performed in controlled laboratory settings using cells or tissues. It is important to note that these products are not categorized as medicines or drugs, and they have not received approval from the FDA for the prevention, treatment, or cure of any medical condition, ailment, or disease. We must emphasize that any form of bodily introduction of these products into humans or animals is strictly prohibited by law. It is essential to adhere to these guidelines to ensure compliance with legal and ethical standards in research and experimentation.