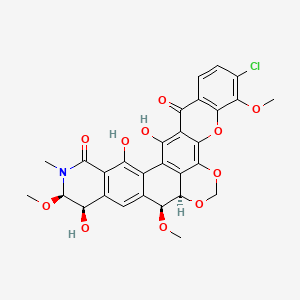
Lysolipin I
Overview
Description
Lysolipin I is a halogenated, polycyclic xanthone antibiotic belonging to the polyketide class, naturally produced by Streptomyces violaceoniger TÜ96 and Streptomyces tendae TÜ4042 . Its molecular formula is C₂₉H₂₄ClNO₁₁, featuring a hexacyclic aromatic core with a pyridone ring and a methoxy substituent at C-24 . This compound exhibits broad-spectrum antibacterial activity, particularly against Gram-positive bacteria, while its efficacy against Gram-negative pathogens is enhanced under conditions of membrane permeability compromise . The compound’s biosynthesis is governed by a 43 kb gene cluster, heterologously expressed in Streptomyces albus to optimize yields up to 1.1 mg/mL in NL800 medium .
Preparation Methods
Synthetic Routes and Reaction Conditions: Lysolipin I is synthesized through a biosynthetic gene cluster spanning 43 kilobases . The heterologous expression of this gene cluster has been established using the cosmid 4H04, which was transferred to Streptomyces albus . Optimization of production yields has been achieved by adapting cultivation conditions and inactivating the transcriptional repressor gene llpRI . Additionally, cultivation in bromide-containing fermentation medium resulted in the production of a new brominated lysolipin derivative .
Industrial Production Methods: Industrial production of this compound involves the use of genetically engineered strains of Streptomyces albus to optimize yields and generate new derivatives . This method ensures consistent production and allows for the creation of derivatives with extended activity against Gram-negative bacteria and reduced cytotoxicity .
Chemical Reactions Analysis
Types of Reactions: Lysolipin I undergoes various chemical reactions, including halogenation, oxidation, and substitution . The presence of a chlorine residue at the xanthone substructure is a notable feature of this compound .
Common Reagents and Conditions: Common reagents used in the reactions involving this compound include bromide for bromination and various solvents such as methylene chloride, dimethyl sulfoxide, and chloroform . The reactions are typically carried out under controlled fermentation conditions to ensure optimal yields .
Major Products Formed: The major products formed from the reactions involving this compound include brominated derivatives and other modified forms with enhanced antibacterial activity and reduced cytotoxicity .
Scientific Research Applications
Lysolipin I has a wide range of scientific research applications, including its use as an antibiotic, antifungal, and anticoccidial agent . It is also a glycopeptide synthesis inhibitor, making it valuable in the study of bacterial cell wall synthesis . Additionally, this compound has shown activity against vancomycin-resistant enterococci and methicillin-resistant Staphylococcus aureus, as well as anaerobic microorganisms such as Helicobacter pylori .
Mechanism of Action
Lysolipin I exerts its effects by inhibiting glycopeptide synthesis, which is crucial for bacterial cell wall formation . The molecular targets of this compound include enzymes involved in the synthesis of peptidoglycan, a key component of the bacterial cell wall . This inhibition disrupts cell wall integrity, leading to bacterial cell death .
Comparison with Similar Compounds
Structural Comparison with Similar Compounds
Lysolipin Derivatives
- Lysolipin X : A chemically unstable precursor that readily converts to Lysolipin I, sharing structural similarities but differing in stability .
- CBS40 (C₂₈H₂₀ClNO₉): Produced by S. albus ∆llpOI, lacks the methoxy group at C-24, resulting in reduced cytotoxicity .
- Brominated Derivative (C₂₈H₂₀BrNO₉): Generated by cultivating S.
- Lysolipin L (C₂₉H₂₂ClNO₁₂) and Lysolipin P (C₂₇H₂₁ClO₁₀): Feature structural variations such as a pyrrolidine ring (instead of piperidine) and altered methylation patterns, impacting bioactivity .
Related Polycyclic Xanthones
- Xantholipin : Shares a hexacyclic xanthone core but exhibits 48% gene cluster similarity to this compound. It lacks the pyridone ring and demonstrates distinct bioactivity profiles .
- Albofungin : Displays 54% gene similarity to this compound and a similar xanthone backbone but differs in post-polyketide modifications .
- FD-594 : A pentangular polyketide with a xanthone ring formed via epoxidation and Baeyer-Villiger oxidation, contrasting this compound’s biosynthetic pathway .
Non-Xanthone Polyketides
- Lysoquinone-TH1 (C₃₃H₂₆O₁₀): A "non-natural" tridecaketide derived from engineered lysolipin pathways. Unlike this compound’s dodecaketide backbone, it inhibits phosphodiesterase 4 (PDE4), relevant for pulmonary diseases, but shows weak antibacterial activity .
- Pradimicin A : Shares a benz[a]napthacene skeleton but includes glycosylation, enhancing antifungal properties absent in this compound .
Bioactivity Comparison
Antimicrobial Activity
Anticancer Activity
- Chloroxanthone Derivatives (e.g., 15d) : Exhibit potent cytotoxicity against MDA-MB-231 cells (IC₅₀ = 0.86 µM), comparable to doxorubicin, via intercalation and topoisomerase inhibition .
- This compound Derivatives : Methylation at C-16 and C-24 is critical for potency; absence reduces activity by 30–50% .
Gene Cluster Similarities
Engineering Strategies
- Transcriptional Repressor Deletion (∆llpRI) : Increases this compound production by3-fold (0.5 g/L → 1.1 mg/mL) .
- Halogenase Gene Knockouts: Enable substitution of chlorine with bromine, altering bioactivity .
- Minimal PKS II Expression: Generates tridecaketides like Lysoquinone-TH1, diverging from native pathways .
Production Yields and Optimization
Biological Activity
Lysolipin I is a halogenated polycyclic xanthone antibiotic produced by the bacterium Streptomyces tendae. This compound has garnered attention due to its potent biological activities, particularly against various bacterial strains and tumor cells. This article provides a comprehensive overview of the biological activity of this compound, including its antibacterial properties, cytotoxic effects, and potential applications in medicine.
Chemical Structure and Properties
This compound is characterized by its complex structure, which includes multiple aromatic rings and halogen substitutions. Its molecular formula is . The presence of chlorine atoms contributes to its unique biological properties, enhancing its interaction with microbial cell membranes.
Antibacterial Activity
This compound exhibits significant antibacterial activity, particularly against Gram-positive bacteria. The Minimum Inhibitory Concentration (MIC) for Gram-positive strains can be as low as , showcasing its potency . In contrast, it also demonstrates some effectiveness against certain Gram-negative bacteria, although the MIC values are generally higher.
Table 1: Antibacterial Activity of this compound
Bacterial Strain | MIC () |
---|---|
Staphylococcus aureus | 0.001 |
Streptococcus pneumoniae | 0.002 |
Escherichia coli | 0.5 |
Pseudomonas aeruginosa | 1.0 |
The mechanism of action for this compound is believed to involve the inhibition of cell wall biosynthesis through interaction with carrier lipids, leading to the accumulation of murein precursors in treated cells . This disruption ultimately results in bacterial cell lysis.
Cytotoxic Effects
In addition to its antibacterial properties, this compound has shown promising cytotoxic effects against various tumor cell lines. The IC50 value for tumor cells has been reported as low as , indicating a strong potential for therapeutic applications in oncology .
Table 2: Cytotoxic Activity of this compound
Tumor Cell Line | IC50 () |
---|---|
HeLa (cervical cancer) | 0.001 |
MCF-7 (breast cancer) | 0.005 |
A549 (lung cancer) | 0.002 |
Case Studies and Research Findings
Recent studies have focused on enhancing the production and derivatives of this compound through genetic engineering techniques. For instance, the deletion of specific regulatory genes in S. albus has resulted in increased yields of this compound production by over 300% . Additionally, structural modifications have been explored to improve antibacterial activity and reduce cytotoxicity.
One notable case involved the creation of a derivative known as CBS40, which exhibited enhanced activity against Escherichia coli while maintaining lower cytotoxicity levels compared to the parent compound . Such findings highlight the potential for developing new antibiotics based on the Lysolipin framework.
Q & A
Basic Research Questions
Q. How is Lysolipin I structurally characterized, and what analytical techniques are essential for its identification?
this compound is characterized using high-resolution electrospray ionization mass spectrometry (HR-ESIMS) and nuclear magnetic resonance (NMR) spectroscopy. HR-ESIMS provides the molecular formula (C29H24NO11Cl) and degrees of unsaturation, while 1D/2D NMR analyses resolve its polycyclic xanthone backbone, chlorination at C-1, and methyl groups at C-16 and C-24. UV-Vis spectroscopy (λmax at 240, 280, 310, 350, and 420 nm) aids in preliminary identification .
Q. What experimental approaches are used to evaluate this compound's antimicrobial activity?
Antimicrobial activity is assessed via minimum inhibitory concentration (MIC) assays against Gram-positive bacteria (e.g., Staphylococcus aureus 8325) and fungi (e.g., Aspergillus spp.). Testing includes dose-response curves and comparison with derivatives to establish structure-activity relationships (SAR). For example, this compound (MIC ≤1 µg/mL) outperforms derivatives lacking methyl groups (e.g., compounds 2 and 3), highlighting the importance of C-16/C-24 methylation .
Q. How are this compound and its derivatives isolated from natural sources?
Isolation involves fermentation of Streptomyces sp. P82B18, followed by chromatographic techniques (e.g., HPLC, LC-MS) to separate polar and non-polar fractions. Non-polar fractions yield aglycons like this compound, while polar fractions contain glycosylated derivatives (e.g., compounds 5–7). Fractionation is guided by UV patterns and molecular weight data .
Advanced Research Questions
Q. What molecular mechanisms underlie this compound's cytotoxicity and DNA synthesis inhibition?
this compound inhibits DNA synthesis by targeting topoisomerase IIβ, as shown in molecular docking studies (PDB 3QX3). It stabilizes DNA-topoisomerase complexes, inducing apoptosis via DNA strand breaks. This mechanism aligns with etoposide, a chemotherapy drug, but this compound's chlorinated xanthone core enhances specificity for microbial and mammalian cell lines .
Q. How do structural modifications (e.g., glycosylation, methylation) impact this compound's bioactivity?
SAR studies reveal that:
- Methylation : Loss of C-16 or C-24 methyl groups reduces cytotoxicity by 20–30% (Figure 37, ).
- Glycosylation : Derivatives with β-D-glucose (e.g., compound 5) exhibit altered retention times and reduced stability due to solvent-mediated methylation (Figure 30, ).
- Chlorination : The C-1 chlorine atom is critical for DNA intercalation, as non-chlorinated analogs show negligible activity .
Q. What challenges arise in elucidating the structures of Lysolipin derivatives, and how are they addressed?
Glycosylated derivatives (e.g., compounds 6–8) present challenges due to low yield, solvent-dependent methylation, and atropisomerization. Strategies include:
- Stable Isotope Labeling : Tracking methylation patterns in deuterated solvents.
- Dynamic HPLC : Resolving atropisomers via temperature-controlled chromatography.
- MS/MS Fragmentation : Differentiating glycosylation sites using collision-induced dissociation (Figure 35, ).
Q. How can contradictions in bioactivity data between this compound and its derivatives be resolved?
Discrepancies often stem from assay conditions (e.g., pH, solvent stability). To mitigate:
- Standardized Protocols : Use uniform MIC assay conditions (e.g., broth microdilution).
- Metabolic Profiling : Monitor derivative stability in culture media via LC-MS.
- Comparative Genomics : Link bioactivity variations to biosynthetic gene cluster (BGC) mutations in Streptomyces strains .
Q. Methodological Guidelines
- For Structural Elucidation : Combine HR-ESIMS, NMR, and X-ray crystallography (if crystals are obtainable). Reference spectral libraries for polycyclic xanthones .
- For Mechanism Studies : Use molecular docking (e.g., AutoDock Vina) with topoisomerase IIβ structures and validate via in vitro DNA relaxation assays .
- For SAR Analysis : Synthesize analogs via targeted gene knockout in Streptomyces or semi-synthetic modification of core structures .
Properties
IUPAC Name |
(7R,8R,12S,13R)-22-chloro-3,8,28-trihydroxy-7,12,21-trimethoxy-6-methyl-14,16,19-trioxa-6-azaheptacyclo[15.11.1.02,11.04,9.013,29.018,27.020,25]nonacosa-1(29),2,4(9),10,17,20(25),21,23,27-nonaene-5,26-dione | |
---|---|---|
Source | PubChem | |
URL | https://pubchem.ncbi.nlm.nih.gov | |
Description | Data deposited in or computed by PubChem | |
InChI |
InChI=1S/C29H24ClNO11/c1-31-28(36)14-10(19(33)29(31)39-4)7-11-13(20(14)34)15-16-25(22(11)37-2)40-8-41-26(16)27-17(21(15)35)18(32)9-5-6-12(30)24(38-3)23(9)42-27/h5-7,19,22,25,29,33-35H,8H2,1-4H3/t19-,22+,25-,29-/m1/s1 | |
Source | PubChem | |
URL | https://pubchem.ncbi.nlm.nih.gov | |
Description | Data deposited in or computed by PubChem | |
InChI Key |
NEOMIZJYHXSRLV-MVHMQXOSSA-N | |
Source | PubChem | |
URL | https://pubchem.ncbi.nlm.nih.gov | |
Description | Data deposited in or computed by PubChem | |
Canonical SMILES |
CN1C(C(C2=C(C1=O)C(=C3C(=C2)C(C4C5=C3C(=C6C(=C5OCO4)OC7=C(C6=O)C=CC(=C7OC)Cl)O)OC)O)O)OC | |
Source | PubChem | |
URL | https://pubchem.ncbi.nlm.nih.gov | |
Description | Data deposited in or computed by PubChem | |
Isomeric SMILES |
CN1[C@@H]([C@@H](C2=C(C1=O)C(=C3C(=C2)[C@@H]([C@H]4C5=C3C(=C6C(=C5OCO4)OC7=C(C6=O)C=CC(=C7OC)Cl)O)OC)O)O)OC | |
Source | PubChem | |
URL | https://pubchem.ncbi.nlm.nih.gov | |
Description | Data deposited in or computed by PubChem | |
Molecular Formula |
C29H24ClNO11 | |
Source | PubChem | |
URL | https://pubchem.ncbi.nlm.nih.gov | |
Description | Data deposited in or computed by PubChem | |
DSSTOX Substance ID |
DTXSID00207855 | |
Record name | Lysolipin I | |
Source | EPA DSSTox | |
URL | https://comptox.epa.gov/dashboard/DTXSID00207855 | |
Description | DSSTox provides a high quality public chemistry resource for supporting improved predictive toxicology. | |
Molecular Weight |
598.0 g/mol | |
Source | PubChem | |
URL | https://pubchem.ncbi.nlm.nih.gov | |
Description | Data deposited in or computed by PubChem | |
CAS No. |
59113-57-4 | |
Record name | Lysolipin I | |
Source | ChemIDplus | |
URL | https://pubchem.ncbi.nlm.nih.gov/substance/?source=chemidplus&sourceid=0059113574 | |
Description | ChemIDplus is a free, web search system that provides access to the structure and nomenclature authority files used for the identification of chemical substances cited in National Library of Medicine (NLM) databases, including the TOXNET system. | |
Record name | Lysolipin I | |
Source | EPA DSSTox | |
URL | https://comptox.epa.gov/dashboard/DTXSID00207855 | |
Description | DSSTox provides a high quality public chemistry resource for supporting improved predictive toxicology. | |
Retrosynthesis Analysis
AI-Powered Synthesis Planning: Our tool employs the Template_relevance Pistachio, Template_relevance Bkms_metabolic, Template_relevance Pistachio_ringbreaker, Template_relevance Reaxys, Template_relevance Reaxys_biocatalysis model, leveraging a vast database of chemical reactions to predict feasible synthetic routes.
One-Step Synthesis Focus: Specifically designed for one-step synthesis, it provides concise and direct routes for your target compounds, streamlining the synthesis process.
Accurate Predictions: Utilizing the extensive PISTACHIO, BKMS_METABOLIC, PISTACHIO_RINGBREAKER, REAXYS, REAXYS_BIOCATALYSIS database, our tool offers high-accuracy predictions, reflecting the latest in chemical research and data.
Strategy Settings
Precursor scoring | Relevance Heuristic |
---|---|
Min. plausibility | 0.01 |
Model | Template_relevance |
Template Set | Pistachio/Bkms_metabolic/Pistachio_ringbreaker/Reaxys/Reaxys_biocatalysis |
Top-N result to add to graph | 6 |
Feasible Synthetic Routes
Disclaimer and Information on In-Vitro Research Products
Please be aware that all articles and product information presented on BenchChem are intended solely for informational purposes. The products available for purchase on BenchChem are specifically designed for in-vitro studies, which are conducted outside of living organisms. In-vitro studies, derived from the Latin term "in glass," involve experiments performed in controlled laboratory settings using cells or tissues. It is important to note that these products are not categorized as medicines or drugs, and they have not received approval from the FDA for the prevention, treatment, or cure of any medical condition, ailment, or disease. We must emphasize that any form of bodily introduction of these products into humans or animals is strictly prohibited by law. It is essential to adhere to these guidelines to ensure compliance with legal and ethical standards in research and experimentation.