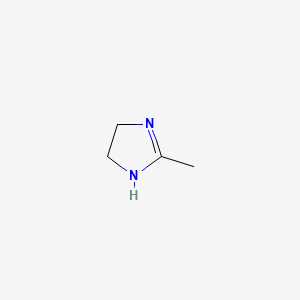
Lysidine
Overview
Description
Lysidine (k2C or L), a hypermodified cytidine derivative, is a critical post-transcriptional modification found at the wobble position (C34) of bacterial tRNA(Ile) with the anticodon CAU . This modification consists of a lysine moiety attached to the C2 position of cytidine via an N-C2 bond, catalyzed by tRNA(Ile)-lysidine synthetase (TilS) in an ATP- and lysine-dependent reaction . This compound serves two essential functions:
- Codon Specificity: Converts the decoding specificity of tRNA(Ile) from AUG (methionine) to AUA (isoleucine) .
- Aminoacylation Specificity: Acts as a determinant for isoleucyl-tRNA synthetase (IleRS), preventing mischarging by methionyl-tRNA synthetase (MetRS) .
Structural studies reveal that the lysine side chain folds back to form a hydrogen bond with the 2′-hydroxyl group of the ribose, stabilizing an anti glycosyl torsion angle (χ = 3°) critical for AUA recognition . This conformation contrasts with unmodified cytidine, which adopts a χ = 33° angle, destabilizing codon-anticodon pairing .
Mechanism of Action
Target of Action
2-Methyl-2-Imidazoline, also known as 2-Methyl-4,5-dihydro-1H-imidazole or Lysidine, interacts with a variety of targets. Some of these include Monomeric sarcosine oxidase, Nicotinate-nucleotide–dimethylbenzimidazole phosphoribosyltransferase, Myoglobin, Nitric oxide synthase, inducible, and Adenylate kinase 2, mitochondrial . These targets play crucial roles in various biological processes, including metabolism and signal transduction .
Mode of Action
It is known to interact with its targets, leading to changes in their activity . For instance, it may inhibit or enhance the activity of enzymes, alter the conformation of proteins, or modulate signal transduction pathways .
Biochemical Pathways
2-Methyl-2-Imidazoline can affect various biochemical pathways due to its interaction with multiple targets . These pathways can include metabolic pathways, signal transduction pathways, and others. The downstream effects of these interactions can lead to changes in cellular functions and responses .
Result of Action
The molecular and cellular effects of 2-Methyl-2-Imidazoline’s action would depend on the specific targets it interacts with and the biochemical pathways it affects . These effects could include changes in enzyme activity, alterations in protein conformation, modulation of signal transduction pathways, and more .
Biochemical Analysis
Biochemical Properties
2-Methyl-2-Imidazoline plays a significant role in biochemical reactions. It interacts with various enzymes, proteins, and other biomolecules . The nature of these interactions is complex and multifaceted, involving a range of biochemical processes .
Cellular Effects
2-Methyl-2-Imidazoline has profound effects on various types of cells and cellular processes. It influences cell function, including impacts on cell signaling pathways, gene expression, and cellular metabolism .
Molecular Mechanism
The mechanism of action of 2-Methyl-2-Imidazoline is intricate. It exerts its effects at the molecular level, including binding interactions with biomolecules, enzyme inhibition or activation, and changes in gene expression .
Temporal Effects in Laboratory Settings
Over time, the effects of 2-Methyl-2-Imidazoline can change in laboratory settings. This includes information on the product’s stability, degradation, and any long-term effects on cellular function observed in in vitro or in vivo studies .
Dosage Effects in Animal Models
The effects of 2-Methyl-2-Imidazoline vary with different dosages in animal models. This includes any threshold effects observed in these studies, as well as any toxic or adverse effects at high doses .
Metabolic Pathways
2-Methyl-2-Imidazoline is involved in several metabolic pathways. It interacts with various enzymes or cofactors, and can also affect metabolic flux or metabolite levels .
Transport and Distribution
2-Methyl-2-Imidazoline is transported and distributed within cells and tissues. It interacts with various transporters or binding proteins, and can also affect its localization or accumulation .
Subcellular Localization
The subcellular localization of 2-Methyl-2-Imidazoline and any effects on its activity or function are complex. This includes any targeting signals or post-translational modifications that direct it to specific compartments or organelles .
Biological Activity
Lysidine, a lysine-containing cytidine derivative, plays a crucial role in the biological activity of bacterial tRNA, particularly in the decoding of the AUA codon. This article explores the synthesis, function, and implications of this compound in various biological contexts, supported by research findings and data tables.
Overview of this compound
This compound is predominantly found at the wobble position (position 34) of tRNA^Ile2 in bacteria. Its presence is essential for the proper recognition and aminoacylation of tRNA by isoleucyl-tRNA synthetase (IleRS), allowing for accurate translation during protein synthesis. The modification converts the codon specificity from AUG (methionine) to AUA (isoleucine), thus influencing the amino acid incorporation during translation .
Synthesis of this compound
The enzyme responsible for this compound synthesis is tRNA^Ile-lysidine synthetase (TilS). The synthesis process involves two main reactions that utilize ATP and lysine as substrates, resulting in an adenylated tRNA intermediate . The structural basis for this enzymatic activity has been elucidated through crystallography studies, revealing insights into the catalytic mechanism of TilS .
The mechanism by which TilS synthesizes this compound includes:
- Recognition : TilS discriminates between tRNA^Ile and tRNA^Met based on structural features of the anticodon loop and acceptor stem .
- Catalysis : The enzyme catalyzes the modification of cytidine to this compound, which is critical for translating AUA codons correctly .
Research Findings
Recent studies have provided valuable insights into the biological significance of this compound. Below are key findings:
Table 1: Kinetic Parameters of TilS Enzyme
Substrate | (μM) | Relative |
---|---|---|
ATP | 1.6 ± 0.5 | 100 |
8-Azido-ATP | 10.0 ± 2.4 | 2.3 |
7-Deaza-ATP | 1.8 ± 0.5 | 8.6 |
N6-Methyl-ATP | 21.7 ± 2.7 | 4.7 |
This table summarizes key kinetic parameters for various nucleotide substrates involved in this compound synthesis, highlighting ATP's role as a primary substrate.
Case Studies
- Mutation Studies : Investigations into mutations within the tilS gene demonstrated that specific alterations could significantly impair this compound formation, emphasizing its critical role in bacterial viability .
- Inhibition Studies : Research has identified several lysine analogs that can inhibit TilS activity, providing potential avenues for antibiotic development targeting bacterial protein synthesis pathways .
Implications for Antibiotic Development
Given that eukaryotic organisms do not possess a homologous enzyme to TilS, it presents a unique target for developing broad-spectrum antibacterial agents. The inhibition of this compound formation could disrupt bacterial protein synthesis without affecting mammalian cells, making it a promising area for therapeutic exploration .
Scientific Research Applications
Synthesis and Function
Lysidine is synthesized by the enzyme tRNA(Ile) this compound synthetase (TilS), which catalyzes the conversion of cytidine to this compound at the wobble position of tRNA(Ile). This modification is critical for determining both the codon specificity (AUA) and amino acid specificity (isoleucine) during protein synthesis . The reaction involves two consecutive steps that utilize ATP and lysine as substrates, forming an adenylated tRNA intermediate .
Role in Codon Recognition
The presence of this compound in tRNA(Ile) allows for specific recognition of the AUA codon, which would otherwise be misread as AUG (methionine). This dual specificity switch is vital for maintaining the fidelity of protein translation in bacterial systems. Studies have shown that without this compound, bacterial strains exhibit reduced efficiency in translating the AUA codon, leading to potential growth defects .
Genetic Information Decoding
This compound's modification of tRNA(Ile) is essential for accurate decoding of genetic information. In Escherichia coli, for instance, the absence of this compound results in misincorporation of methionine instead of isoleucine when translating AUA codons. This alteration can significantly affect cellular functions and viability . The tilS gene responsible for this compound synthesis is conserved across many bacterial species, indicating its fundamental role in bacterial physiology .
Potential as a Target for Antibiotics
Given its critical role in bacterial translation, this compound synthesis pathways represent potential targets for novel antibiotic development. Inhibitors that disrupt TilS function could selectively impair protein synthesis in pathogenic bacteria without affecting eukaryotic cells, offering a promising avenue for antibiotic therapy .
Antimicrobial Strategies
Research has indicated that manipulating this compound pathways may enhance antimicrobial strategies. For example, understanding how this compound affects tRNA specificity could lead to engineered tRNAs that improve the efficacy of antimicrobial peptides or other therapeutic agents against resistant bacterial strains .
Biotechnological Applications
This compound-modified tRNAs could be utilized in synthetic biology to create organisms with tailored protein synthesis capabilities. By incorporating this compound into engineered tRNAs, researchers can expand the genetic code to include non-canonical amino acids or enhance protein production systems .
Case Studies
Q & A
Q. Basic: What experimental methods are used to determine the structural basis of lysidine's role in tRNA specificity?
Answer:
this compound's structural role in tRNA(Ile) specificity can be elucidated using X-ray crystallography to resolve the 3D structure of TilS (tRNA(Ile) this compound synthetase) complexed with tRNA. For example, the crystal structure of Aquifex aeolicus TilS at 2.42 Å resolution revealed a homodimeric architecture with distinct domains for adenylation and lysine incorporation . Mutagenesis studies targeting conserved residues (e.g., catalytic loop regions) further validate functional sites. Complementary kinetic assays (e.g., measuring ATP consumption or this compound formation rates) can confirm enzymatic activity .
Q. Basic: How is this compound detected and quantified in tRNA samples?
Answer:
this compound detection requires high-performance liquid chromatography (HPLC) coupled with mass spectrometry (MS) . For example, tRNA hydrolysates are separated via reverse-phase HPLC, and this compound is identified by its unique mass-to-charge ratio (m/z = 255.1 for protonated this compound). Quantification is achieved using synthetic this compound standards . NMR spectroscopy can also confirm this compound’s chemical structure in purified tRNA fractions by analyzing characteristic imidazoline ring signals .
Q. Advanced: How can researchers design experiments to investigate the catalytic mechanism of TilS?
Answer:
A robust approach combines pre-steady-state kinetics and structural biology :
Kinetic isotope effects (KIE) : Use isotopically labeled ATP (e.g., γ-¹⁸O-ATP) to track adenylation steps.
Time-resolved crystallography : Capture intermediate states of TilS during catalysis (e.g., ATP-bound vs. This compound-bound conformers) .
Site-directed mutagenesis : Replace conserved residues (e.g., Arg/Lys in the active site) to disrupt substrate binding or catalysis. Measure activity loss via radiolabeled lysine incorporation assays .
Molecular dynamics simulations : Model substrate-induced conformational changes in TilS to predict transition states .
Q. Advanced: How can contradictions in this compound’s stability under varying acidic conditions be resolved?
Answer:
Conflicting data on this compound hydrolysis rates (e.g., in HCl vs. H₂SO₄) arise from differences in acid strength measurement (e.g., H₀ vs. Hₐᴍ scales) and water activity . To resolve discrepancies:
Standardize acid concentration metrics : Use Hammett acidity functions (H₀) for concentrated H₂SO₄ and pH meters for dilute HCl.
Control water activity : Add inert salts (e.g., LiCl) to maintain consistent ionic strength .
Monitor intermediates : Use ¹H NMR to detect transient species (e.g., lysidinium dications) and validate proposed hydrolysis pathways .
Q. Advanced: What strategies distinguish this compound-modified tRNA(Ile) from unmodified tRNA(Met) in structural studies?
Answer:
Cryo-electron microscopy (cryo-EM) can differentiate tRNA(Ile) and tRNA(Met) based on anticodon-loop conformation. Additionally:
Chemical probing : Treat tRNA with dimethyl sulfate (DMS) , which methylates unpaired cytosines. This compound’s modified C34 resists methylation, confirmed via primer extension assays .
Selective enzymatic digestion : Use RNase T1 , which cleaves unmodified cytidine residues but not this compound, to isolate tRNA(Ile) .
Comparative genomics : Identify conserved TilS-binding motifs (e.g., C29•G41 base pair in A. aeolicus tRNA(Ile)) via sequence alignment .
Q. Advanced: How do this compound and acetylated cytidine (ac4C) collaborate to maintain translational fidelity?
Answer:
Cooperative roles are validated via genetic interaction studies :
Knockout strains : Delete tilS (this compound synthesis) and tmcAL (ac4C synthesis) in E. coli. Measure misincorporation rates (e.g., methionine at AUA codons) via LC-MS/MS proteomics .
Structural analysis : Solve ribosome structures with tRNA(Ile) to visualize ac4C-lysidine stacking interactions stabilizing codon-anticodon pairing .
Biochemical assays : Compare aminoacylation efficiency of tRNA(Ile) with/without ac4C and this compound using radioactive isoleucine incorporation .
Comparison with Similar Compounds
Lysidine vs. Cytidine
This compound’s lysine moiety sterically blocks AUG decoding while enabling AUA specificity through tautomerization, where the N3 and O2 positions form hydrogen bonds with adenine . Cytidine lacks this modification, leading to methionine misincorporation at AUA codons .
This compound vs. Agmatidine (agm2C)
Agmatidine, found in archaeal tRNA(Ile), is a cytidine derivative modified with agmatine (decarboxylated arginine) at C2. Despite functional similarities, key differences exist:
Both modifications prevent AUG recognition by excluding guanine through steric hindrance . However, TilS and TiaS exhibit distinct tRNA recognition strategies: TilS targets the acceptor stem (e.g., C4-G69 in E. coli), while TiaS recognizes the anticodon stem .
This compound vs. Unmodified tRNA(Met)
tRNA(Ile) and tRNA(Met) share identical anticodon sequences (CAU) but are distinguished by this compound modification:
TilS discriminates tRNA(Ile) from tRNA(Met) by recognizing conserved base pairs in the acceptor stem, ensuring fidelity in AUA decoding . Loss of this compound (e.g., in TilS mutants) reduces isoleucine incorporation by >90%, leading to mistranslation .
Structural and Functional Insights from Quantum Chemical Studies
- Conformational Stability : this compound’s 5′-3′ diphosphate backbone stabilizes zwitterionic and tautomeric forms, enhancing ribose puckering (C3′-endo) and AUA recognition .
- Tautomerization: The tautomeric form of this compound donates and accepts H-bonds with adenine, mimicking guanosine’s pairing pattern .
- Enzyme Flexibility : TilS can utilize lysine analogs (e.g., ornithine) to generate alternative modifications, though IleRS preferentially recognizes this compound .
Evolutionary and Biomedical Significance
- Divergent Evolution : this compound (bacteria) and agmatidine (archaea) represent convergent solutions to AUA decoding, highlighting domain-specific adaptation .
- Drug Target Potential: Absence of this compound in humans makes TilS a promising target for antibacterial and antimalarial therapies .
Tables
Table 1. Key Structural Parameters of this compound vs. Cytidine
Parameter | This compound (k2C) | Cytidine (C) |
---|---|---|
Glycosyl Torsion | 3° (anti) | 33° |
Pseudorotation (P) | 18° (C3′-endo) | 38° (C2′-endo) |
H-bond Network | Lysine-2′-OH | None |
Table 2. Enzymatic Comparison: TilS vs. TiaS
Enzyme | Substrate | Recognition Site | Domain Organization |
---|---|---|---|
TilS | tRNA(Ile) | Acceptor stem (C4-G69) | N-terminal ATPase, C-terminal tRNA-binding |
TiaS | tRNA(Ile) | Anticodon stem | ATPase + agmatine-binding |
Properties
IUPAC Name |
2-methyl-4,5-dihydro-1H-imidazole | |
---|---|---|
Source | PubChem | |
URL | https://pubchem.ncbi.nlm.nih.gov | |
Description | Data deposited in or computed by PubChem | |
InChI |
InChI=1S/C4H8N2/c1-4-5-2-3-6-4/h2-3H2,1H3,(H,5,6) | |
Source | PubChem | |
URL | https://pubchem.ncbi.nlm.nih.gov | |
Description | Data deposited in or computed by PubChem | |
InChI Key |
VWSLLSXLURJCDF-UHFFFAOYSA-N | |
Source | PubChem | |
URL | https://pubchem.ncbi.nlm.nih.gov | |
Description | Data deposited in or computed by PubChem | |
Canonical SMILES |
CC1=NCCN1 | |
Source | PubChem | |
URL | https://pubchem.ncbi.nlm.nih.gov | |
Description | Data deposited in or computed by PubChem | |
Molecular Formula |
C4H8N2 | |
Source | PubChem | |
URL | https://pubchem.ncbi.nlm.nih.gov | |
Description | Data deposited in or computed by PubChem | |
DSSTOX Substance ID |
DTXSID2060204 | |
Record name | 1H-Imidazole, 4,5-dihydro-2-methyl- | |
Source | EPA DSSTox | |
URL | https://comptox.epa.gov/dashboard/DTXSID2060204 | |
Description | DSSTox provides a high quality public chemistry resource for supporting improved predictive toxicology. | |
Molecular Weight |
84.12 g/mol | |
Source | PubChem | |
URL | https://pubchem.ncbi.nlm.nih.gov | |
Description | Data deposited in or computed by PubChem | |
CAS No. |
534-26-9 | |
Record name | 2-Methylimidazoline | |
Source | CAS Common Chemistry | |
URL | https://commonchemistry.cas.org/detail?cas_rn=534-26-9 | |
Description | CAS Common Chemistry is an open community resource for accessing chemical information. Nearly 500,000 chemical substances from CAS REGISTRY cover areas of community interest, including common and frequently regulated chemicals, and those relevant to high school and undergraduate chemistry classes. This chemical information, curated by our expert scientists, is provided in alignment with our mission as a division of the American Chemical Society. | |
Explanation | The data from CAS Common Chemistry is provided under a CC-BY-NC 4.0 license, unless otherwise stated. | |
Record name | Lysidine | |
Source | ChemIDplus | |
URL | https://pubchem.ncbi.nlm.nih.gov/substance/?source=chemidplus&sourceid=0000534269 | |
Description | ChemIDplus is a free, web search system that provides access to the structure and nomenclature authority files used for the identification of chemical substances cited in National Library of Medicine (NLM) databases, including the TOXNET system. | |
Record name | 1H-Imidazole, 4,5-dihydro-2-methyl- | |
Source | EPA Chemicals under the TSCA | |
URL | https://www.epa.gov/chemicals-under-tsca | |
Description | EPA Chemicals under the Toxic Substances Control Act (TSCA) collection contains information on chemicals and their regulations under TSCA, including non-confidential content from the TSCA Chemical Substance Inventory and Chemical Data Reporting. | |
Record name | 1H-Imidazole, 4,5-dihydro-2-methyl- | |
Source | EPA DSSTox | |
URL | https://comptox.epa.gov/dashboard/DTXSID2060204 | |
Description | DSSTox provides a high quality public chemistry resource for supporting improved predictive toxicology. | |
Record name | 4,5-dihydro-2-methyl-1H-imidazole | |
Source | European Chemicals Agency (ECHA) | |
URL | https://echa.europa.eu/substance-information/-/substanceinfo/100.007.816 | |
Description | The European Chemicals Agency (ECHA) is an agency of the European Union which is the driving force among regulatory authorities in implementing the EU's groundbreaking chemicals legislation for the benefit of human health and the environment as well as for innovation and competitiveness. | |
Explanation | Use of the information, documents and data from the ECHA website is subject to the terms and conditions of this Legal Notice, and subject to other binding limitations provided for under applicable law, the information, documents and data made available on the ECHA website may be reproduced, distributed and/or used, totally or in part, for non-commercial purposes provided that ECHA is acknowledged as the source: "Source: European Chemicals Agency, http://echa.europa.eu/". Such acknowledgement must be included in each copy of the material. ECHA permits and encourages organisations and individuals to create links to the ECHA website under the following cumulative conditions: Links can only be made to webpages that provide a link to the Legal Notice page. | |
Record name | LYSIDINE | |
Source | FDA Global Substance Registration System (GSRS) | |
URL | https://gsrs.ncats.nih.gov/ginas/app/beta/substances/987F50E3PE | |
Description | The FDA Global Substance Registration System (GSRS) enables the efficient and accurate exchange of information on what substances are in regulated products. Instead of relying on names, which vary across regulatory domains, countries, and regions, the GSRS knowledge base makes it possible for substances to be defined by standardized, scientific descriptions. | |
Explanation | Unless otherwise noted, the contents of the FDA website (www.fda.gov), both text and graphics, are not copyrighted. They are in the public domain and may be republished, reprinted and otherwise used freely by anyone without the need to obtain permission from FDA. Credit to the U.S. Food and Drug Administration as the source is appreciated but not required. | |
Retrosynthesis Analysis
AI-Powered Synthesis Planning: Our tool employs the Template_relevance Pistachio, Template_relevance Bkms_metabolic, Template_relevance Pistachio_ringbreaker, Template_relevance Reaxys, Template_relevance Reaxys_biocatalysis model, leveraging a vast database of chemical reactions to predict feasible synthetic routes.
One-Step Synthesis Focus: Specifically designed for one-step synthesis, it provides concise and direct routes for your target compounds, streamlining the synthesis process.
Accurate Predictions: Utilizing the extensive PISTACHIO, BKMS_METABOLIC, PISTACHIO_RINGBREAKER, REAXYS, REAXYS_BIOCATALYSIS database, our tool offers high-accuracy predictions, reflecting the latest in chemical research and data.
Strategy Settings
Precursor scoring | Relevance Heuristic |
---|---|
Min. plausibility | 0.01 |
Model | Template_relevance |
Template Set | Pistachio/Bkms_metabolic/Pistachio_ringbreaker/Reaxys/Reaxys_biocatalysis |
Top-N result to add to graph | 6 |
Feasible Synthetic Routes
Disclaimer and Information on In-Vitro Research Products
Please be aware that all articles and product information presented on BenchChem are intended solely for informational purposes. The products available for purchase on BenchChem are specifically designed for in-vitro studies, which are conducted outside of living organisms. In-vitro studies, derived from the Latin term "in glass," involve experiments performed in controlled laboratory settings using cells or tissues. It is important to note that these products are not categorized as medicines or drugs, and they have not received approval from the FDA for the prevention, treatment, or cure of any medical condition, ailment, or disease. We must emphasize that any form of bodily introduction of these products into humans or animals is strictly prohibited by law. It is essential to adhere to these guidelines to ensure compliance with legal and ethical standards in research and experimentation.