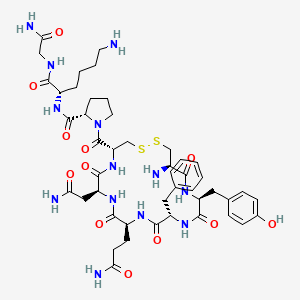
Lypressin
Overview
Description
Lypressin is a synthetic polypeptide with vasoconstrictor and antidiuretic effects . It is used to prevent or control the frequent urination, increased thirst, and loss of water associated with diabetes insipidus . Its molecular weight is 1056.2 Dalton and the molecular formula is C46H65N13O12S2 .
Synthesis Analysis
Human neurohormone vasopressin (AVP), which is similar to Lypressin, is synthesized in overlapping regions in the hypothalamus . It is mainly known for its vasoconstricting abilities, and it is responsible for the regulation of plasma osmolality by maintaining fluid homeostasis .Molecular Structure Analysis
The molecular structure of Lypressin is C46H65N13O12S2 . It has a weight average of 1056.23 and a mono-isotopic mass of 1055.431706933 .Physical And Chemical Properties Analysis
Lypressin has a molecular formula of C46H65N13O12S2 and an average mass of 1056.218 Da . It has a density of 1.3±0.1 g/cm3, a boiling point of 1610.4±65.0 °C at 760 mmHg, and a vapour pressure of 0.0±0.3 mmHg at 25°C .Scientific Research Applications
Pain Management
Lypressin, a vasopressin analogue, has been studied for its potential analgesic properties . However, the research indicated that Lypressin did not have any marked analgesic effect on ischaemic, cutaneous thermal, or dental pain at doses low enough not to produce a hazardous water retention .
Fluid Homeostasis
Lypressin is an analogue of the human neurohormone vasopressin (AVP), which is synthesized in overlapping regions in the hypothalamus . AVP is primarily known for its vasoconstricting abilities and is responsible for the regulation of plasma osmolality by maintaining fluid homeostasis .
Treatment of Diabetes Insipidus
Lypressin has been successfully applied as a therapeutic agent in the treatment of diabetes insipidus . Diabetes insipidus is a condition characterized by excessive thirst and excretion of large amounts of severely diluted urine.
Cardiovascular Pressor Response
Vasopressin, the hormone that Lypressin is an analogue of, is known to have a cardiovascular pressor response . This means it can cause the constriction of blood vessels, which increases blood pressure.
Potential Treatment for SARS-CoV-2
Recent research has discussed the potential application of Lypressin in the treatment of SARS-CoV-2 . However, more research is needed to confirm its efficacy and safety in this application.
Research Tool in Pharmacology
Lypressin is used as a research tool in pharmacology, particularly in studies investigating the properties and effects of vasopressin .
Mechanism of Action
Target of Action
Lypressin, also known as 8-L-lysinevasopressin, primarily targets the Vasopressin V1a, V1b, and V2 receptors . These receptors play a crucial role in maintaining fluid homeostasis and vasoconstriction .
Mode of Action
Lypressin interacts with its targets, the Vasopressin receptors, to exert its effects . It increases cyclic adenosine monophosphate (cAMP), which in turn increases water permeability at the renal tubule, resulting in decreased urine volume and increased osmolality . Lypressin also causes peristalsis by directly stimulating the smooth muscle in the gastrointestinal tract .
Biochemical Pathways
It is known that lypressin’s interaction with the vasopressin receptors leads to an increase in camp . This increase in cAMP enhances water permeability in the renal tubules, affecting the water reabsorption pathway .
Pharmacokinetics
It is known that lypressin is a prodrug of lysine-vasopressin, which has a longer half-life and increased selectivity for the v1 receptor compared to endogenous vasopressin .
Result of Action
The primary result of Lypressin’s action is the reduction of urine volume and increase in urine osmolality due to enhanced water reabsorption in the renal tubules . This can help manage conditions like central diabetes insipidus . Additionally, Lypressin’s stimulation of smooth muscle in the gastrointestinal tract can promote peristalsis .
Safety and Hazards
Lypressin is contraindicated in chronic nephritis with nitrogen retention . Special precautions should be taken in cases of hypertension or conditions which can be exacerbated by increased BP; nasal absorption may be impaired in rhinitis . Adverse reactions include nasal congestion, irritation, ulceration, hypersensitivity reactions, and in large doses, marked pallor, pounding headache, vertigo, sweating, tremor, GI disturbances, eructation, cramp, desire to defaecate, and hyponatraemia .
properties
IUPAC Name |
(2S)-N-[(2S)-6-amino-1-[(2-amino-2-oxoethyl)amino]-1-oxohexan-2-yl]-1-[(4R,7S,10S,13S,16S,19R)-19-amino-7-(2-amino-2-oxoethyl)-10-(3-amino-3-oxopropyl)-13-benzyl-16-[(4-hydroxyphenyl)methyl]-6,9,12,15,18-pentaoxo-1,2-dithia-5,8,11,14,17-pentazacycloicosane-4-carbonyl]pyrrolidine-2-carboxamide | |
---|---|---|
Source | PubChem | |
URL | https://pubchem.ncbi.nlm.nih.gov | |
Description | Data deposited in or computed by PubChem | |
InChI |
InChI=1S/C46H65N13O12S2/c47-17-5-4-9-29(40(65)52-22-38(51)63)54-45(70)35-10-6-18-59(35)46(71)34-24-73-72-23-28(48)39(64)55-31(20-26-11-13-27(60)14-12-26)43(68)56-32(19-25-7-2-1-3-8-25)42(67)53-30(15-16-36(49)61)41(66)57-33(21-37(50)62)44(69)58-34/h1-3,7-8,11-14,28-35,60H,4-6,9-10,15-24,47-48H2,(H2,49,61)(H2,50,62)(H2,51,63)(H,52,65)(H,53,67)(H,54,70)(H,55,64)(H,56,68)(H,57,66)(H,58,69)/t28-,29-,30-,31-,32-,33-,34-,35-/m0/s1 | |
Source | PubChem | |
URL | https://pubchem.ncbi.nlm.nih.gov | |
Description | Data deposited in or computed by PubChem | |
InChI Key |
BJFIDCADFRDPIO-DZCXQCEKSA-N | |
Source | PubChem | |
URL | https://pubchem.ncbi.nlm.nih.gov | |
Description | Data deposited in or computed by PubChem | |
Canonical SMILES |
C1CC(N(C1)C(=O)C2CSSCC(C(=O)NC(C(=O)NC(C(=O)NC(C(=O)NC(C(=O)N2)CC(=O)N)CCC(=O)N)CC3=CC=CC=C3)CC4=CC=C(C=C4)O)N)C(=O)NC(CCCCN)C(=O)NCC(=O)N | |
Source | PubChem | |
URL | https://pubchem.ncbi.nlm.nih.gov | |
Description | Data deposited in or computed by PubChem | |
Isomeric SMILES |
C1C[C@H](N(C1)C(=O)[C@@H]2CSSC[C@@H](C(=O)N[C@H](C(=O)N[C@H](C(=O)N[C@H](C(=O)N[C@H](C(=O)N2)CC(=O)N)CCC(=O)N)CC3=CC=CC=C3)CC4=CC=C(C=C4)O)N)C(=O)N[C@@H](CCCCN)C(=O)NCC(=O)N | |
Source | PubChem | |
URL | https://pubchem.ncbi.nlm.nih.gov | |
Description | Data deposited in or computed by PubChem | |
Molecular Formula |
C46H65N13O12S2 | |
Source | PubChem | |
URL | https://pubchem.ncbi.nlm.nih.gov | |
Description | Data deposited in or computed by PubChem | |
DSSTOX Substance ID |
DTXSID8048575 | |
Record name | Lypressin | |
Source | EPA DSSTox | |
URL | https://comptox.epa.gov/dashboard/DTXSID8048575 | |
Description | DSSTox provides a high quality public chemistry resource for supporting improved predictive toxicology. | |
Molecular Weight |
1056.2 g/mol | |
Source | PubChem | |
URL | https://pubchem.ncbi.nlm.nih.gov | |
Description | Data deposited in or computed by PubChem | |
Product Name |
Lypressin | |
CAS RN |
50-57-7 | |
Record name | Lypressin [USAN:USP:INN:BAN] | |
Source | ChemIDplus | |
URL | https://pubchem.ncbi.nlm.nih.gov/substance/?source=chemidplus&sourceid=0000050577 | |
Description | ChemIDplus is a free, web search system that provides access to the structure and nomenclature authority files used for the identification of chemical substances cited in National Library of Medicine (NLM) databases, including the TOXNET system. | |
Record name | Lypressin | |
Source | DrugBank | |
URL | https://www.drugbank.ca/drugs/DB14642 | |
Description | The DrugBank database is a unique bioinformatics and cheminformatics resource that combines detailed drug (i.e. chemical, pharmacological and pharmaceutical) data with comprehensive drug target (i.e. sequence, structure, and pathway) information. | |
Explanation | Creative Common's Attribution-NonCommercial 4.0 International License (http://creativecommons.org/licenses/by-nc/4.0/legalcode) | |
Record name | Lypressin | |
Source | EPA DSSTox | |
URL | https://comptox.epa.gov/dashboard/DTXSID8048575 | |
Description | DSSTox provides a high quality public chemistry resource for supporting improved predictive toxicology. | |
Record name | Lypressin | |
Source | European Chemicals Agency (ECHA) | |
URL | https://echa.europa.eu/substance-information/-/substanceinfo/100.000.046 | |
Description | The European Chemicals Agency (ECHA) is an agency of the European Union which is the driving force among regulatory authorities in implementing the EU's groundbreaking chemicals legislation for the benefit of human health and the environment as well as for innovation and competitiveness. | |
Explanation | Use of the information, documents and data from the ECHA website is subject to the terms and conditions of this Legal Notice, and subject to other binding limitations provided for under applicable law, the information, documents and data made available on the ECHA website may be reproduced, distributed and/or used, totally or in part, for non-commercial purposes provided that ECHA is acknowledged as the source: "Source: European Chemicals Agency, http://echa.europa.eu/". Such acknowledgement must be included in each copy of the material. ECHA permits and encourages organisations and individuals to create links to the ECHA website under the following cumulative conditions: Links can only be made to webpages that provide a link to the Legal Notice page. | |
Retrosynthesis Analysis
AI-Powered Synthesis Planning: Our tool employs the Template_relevance Pistachio, Template_relevance Bkms_metabolic, Template_relevance Pistachio_ringbreaker, Template_relevance Reaxys, Template_relevance Reaxys_biocatalysis model, leveraging a vast database of chemical reactions to predict feasible synthetic routes.
One-Step Synthesis Focus: Specifically designed for one-step synthesis, it provides concise and direct routes for your target compounds, streamlining the synthesis process.
Accurate Predictions: Utilizing the extensive PISTACHIO, BKMS_METABOLIC, PISTACHIO_RINGBREAKER, REAXYS, REAXYS_BIOCATALYSIS database, our tool offers high-accuracy predictions, reflecting the latest in chemical research and data.
Strategy Settings
Precursor scoring | Relevance Heuristic |
---|---|
Min. plausibility | 0.01 |
Model | Template_relevance |
Template Set | Pistachio/Bkms_metabolic/Pistachio_ringbreaker/Reaxys/Reaxys_biocatalysis |
Top-N result to add to graph | 6 |
Feasible Synthetic Routes
Disclaimer and Information on In-Vitro Research Products
Please be aware that all articles and product information presented on BenchChem are intended solely for informational purposes. The products available for purchase on BenchChem are specifically designed for in-vitro studies, which are conducted outside of living organisms. In-vitro studies, derived from the Latin term "in glass," involve experiments performed in controlled laboratory settings using cells or tissues. It is important to note that these products are not categorized as medicines or drugs, and they have not received approval from the FDA for the prevention, treatment, or cure of any medical condition, ailment, or disease. We must emphasize that any form of bodily introduction of these products into humans or animals is strictly prohibited by law. It is essential to adhere to these guidelines to ensure compliance with legal and ethical standards in research and experimentation.