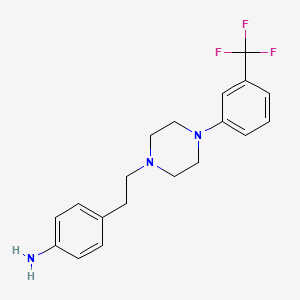
Papp
Overview
Description
LY 165,163 is a chemical compound known for its activity as a partial agonist of serotonin receptors 5-HT1A and 5-HT1D. It also exhibits marked activity at both pre- and postsynaptic dopaminergic D2 (D3 and D1) receptors . This compound has been studied for its potential therapeutic applications, including the treatment of ocular pain .
Mechanism of Action
Target of Action
Pregnancy-associated plasma protein-A (PAPP-A) is a protein that plays an integral role in various biological processes, particularly in breast cancer (BC), especially triple negative breast cancer (TNBC) . It belongs to the pappalysin subfamily . The primary targets of this compound-A are insulin-like growth factor-binding proteins (IGFBPs), specifically IGFBP-4 .
Mode of Action
This compound-A is a metzincin metalloproteinase that regulates local insulin-like growth factor (IGF) activity through the cleavage of high-affinity IGFBPs .
Biochemical Pathways
This compound-A is heavily involved in p53 mediated pathways, collagen deposition, epithelial-mesenchymal transition (EMT), and extracellular matrix (ECM) tissue remodeling of BCs . It plays a crucial role in the IGFBP/IGF axis, which is important for cell proliferation, differentiation, migration, and survival .
Pharmacokinetics
Membrane permeability is an in vitro parameter that represents the apparent permeability (this compound) of a compound, and is a key absorption, distribution, metabolism, and excretion (ADME) parameter in drug development . .
Result of Action
The proteolytic regulation of IGF activity by this compound-A is important, as the IGFs promote proliferation, differentiation, migration, and survival in various normal and cancer cells . In breast cancer, this compound-A has been demonstrated to play a role in tumor initiation, progression, metastasis including EMT, as well as acting as a biomarker for predicting patient outcomes .
Action Environment
The action of this compound-A is influenced by various environmental factors. For instance, during pregnancy, the placental syncytiotrophoblasts synthesize and secrete this compound-A into the plasma and maintain its high levels . .
Biochemical Analysis
Biochemical Properties
PAPP-A is involved in the regulation of Insulin-like Growth Factor (IGF) availability through its specific proteolytic activity against IGF Binding Protein 4 (IGFBP-4) . This interaction between this compound-A and IGFBP-4 is crucial for the modulation of IGF signaling pathways .
Cellular Effects
This compound-A influences cell function by modulating the IGF signaling pathways, which are critical for cell growth, differentiation, and survival . Changes in this compound-A levels can impact these cellular processes, potentially leading to complications such as pre-eclampsia .
Molecular Mechanism
The molecular mechanism of this compound-A involves its proteolytic activity toward IGFBP-4, which requires the cooperation of two subunits of the this compound-A dimer . This proteolytic activity releases IGFs, allowing them to bind to their receptors and exert their effects .
Temporal Effects in Laboratory Settings
The effects of this compound-A in laboratory settings can vary over time. For instance, low levels of this compound-A in the first trimester of pregnancy have been associated with a higher incidence of pre-eclampsia .
Metabolic Pathways
This compound-A is involved in the IGF signaling pathway, a critical metabolic pathway that regulates growth and development . It interacts with IGFBP-4 to modulate the availability of IGFs .
Transport and Distribution
This compound-A is produced in the placenta and released into the maternal bloodstream , indicating that it is transported and distributed through the circulatory system.
Preparation Methods
The synthesis of LY 165,163 involves the substitution of piperazine by 2-(4-aminophenyl)ethyl and 3-(trifluoromethyl)phenyl groups at positions 1 and 4, respectively . The synthetic route typically involves the following steps:
Formation of the piperazine core: This involves the reaction of appropriate amines with piperazine.
Substitution reactions: The piperazine core is then substituted with 2-(4-aminophenyl)ethyl and 3-(trifluoromethyl)phenyl groups under specific reaction conditions.
Chemical Reactions Analysis
LY 165,163 undergoes various chemical reactions, including:
Oxidation: This compound can be oxidized under specific conditions to form corresponding oxidized products.
Reduction: LY 165,163 can be reduced to form reduced derivatives.
Substitution: The compound can undergo substitution reactions, particularly at the piperazine core and the aromatic rings.
Common reagents and conditions used in these reactions include oxidizing agents like potassium permanganate, reducing agents like sodium borohydride, and various catalysts for substitution reactions . The major products formed from these reactions depend on the specific conditions and reagents used.
Scientific Research Applications
LY 165,163 has several scientific research applications, including:
Comparison with Similar Compounds
LY 165,163 is unique in its dual activity on both serotonin and dopamine receptors. Similar compounds include:
1-(3-(trifluoromethyl)phenyl)piperazine: This compound shares structural similarities with LY 165,163 and also acts on serotonin receptors.
4-(2-(4-(3-(trifluoromethyl)phenyl)-1-piperazinyl)ethyl)benzenamine: Another structurally related compound with activity on serotonin receptors.
The uniqueness of LY 165,163 lies in its combined activity on both serotonin and dopamine receptors, which makes it a valuable compound for research and potential therapeutic applications .
Properties
IUPAC Name |
4-[2-[4-[3-(trifluoromethyl)phenyl]piperazin-1-yl]ethyl]aniline | |
---|---|---|
Source | PubChem | |
URL | https://pubchem.ncbi.nlm.nih.gov | |
Description | Data deposited in or computed by PubChem | |
InChI |
InChI=1S/C19H22F3N3/c20-19(21,22)16-2-1-3-18(14-16)25-12-10-24(11-13-25)9-8-15-4-6-17(23)7-5-15/h1-7,14H,8-13,23H2 | |
Source | PubChem | |
URL | https://pubchem.ncbi.nlm.nih.gov | |
Description | Data deposited in or computed by PubChem | |
InChI Key |
GAAKALASJNGQKD-UHFFFAOYSA-N | |
Source | PubChem | |
URL | https://pubchem.ncbi.nlm.nih.gov | |
Description | Data deposited in or computed by PubChem | |
Canonical SMILES |
C1CN(CCN1CCC2=CC=C(C=C2)N)C3=CC=CC(=C3)C(F)(F)F | |
Source | PubChem | |
URL | https://pubchem.ncbi.nlm.nih.gov | |
Description | Data deposited in or computed by PubChem | |
Molecular Formula |
C19H22F3N3 | |
Source | PubChem | |
URL | https://pubchem.ncbi.nlm.nih.gov | |
Description | Data deposited in or computed by PubChem | |
DSSTOX Substance ID |
DTXSID30171136 | |
Record name | 4-(2-(4-(3-(Trifluoromethyl)phenyl)-1-piperazinyl)ethyl)-Benzenamine | |
Source | EPA DSSTox | |
URL | https://comptox.epa.gov/dashboard/DTXSID30171136 | |
Description | DSSTox provides a high quality public chemistry resource for supporting improved predictive toxicology. | |
Molecular Weight |
349.4 g/mol | |
Source | PubChem | |
URL | https://pubchem.ncbi.nlm.nih.gov | |
Description | Data deposited in or computed by PubChem | |
Solubility |
>52.4 [ug/mL] (The mean of the results at pH 7.4) | |
Record name | SID46500433 | |
Source | Burnham Center for Chemical Genomics | |
URL | https://pubchem.ncbi.nlm.nih.gov/bioassay/1996#section=Data-Table | |
Description | Aqueous solubility in buffer at pH 7.4 | |
CAS No. |
1814-64-8 | |
Record name | LY 165163 | |
Source | ChemIDplus | |
URL | https://pubchem.ncbi.nlm.nih.gov/substance/?source=chemidplus&sourceid=0001814648 | |
Description | ChemIDplus is a free, web search system that provides access to the structure and nomenclature authority files used for the identification of chemical substances cited in National Library of Medicine (NLM) databases, including the TOXNET system. | |
Record name | 4-(2-(4-(3-(Trifluoromethyl)phenyl)-1-piperazinyl)ethyl)-Benzenamine | |
Source | EPA DSSTox | |
URL | https://comptox.epa.gov/dashboard/DTXSID30171136 | |
Description | DSSTox provides a high quality public chemistry resource for supporting improved predictive toxicology. | |
Record name | LY-165163 | |
Source | FDA Global Substance Registration System (GSRS) | |
URL | https://gsrs.ncats.nih.gov/ginas/app/beta/substances/8HAJ699EWG | |
Description | The FDA Global Substance Registration System (GSRS) enables the efficient and accurate exchange of information on what substances are in regulated products. Instead of relying on names, which vary across regulatory domains, countries, and regions, the GSRS knowledge base makes it possible for substances to be defined by standardized, scientific descriptions. | |
Explanation | Unless otherwise noted, the contents of the FDA website (www.fda.gov), both text and graphics, are not copyrighted. They are in the public domain and may be republished, reprinted and otherwise used freely by anyone without the need to obtain permission from FDA. Credit to the U.S. Food and Drug Administration as the source is appreciated but not required. | |
Retrosynthesis Analysis
AI-Powered Synthesis Planning: Our tool employs the Template_relevance Pistachio, Template_relevance Bkms_metabolic, Template_relevance Pistachio_ringbreaker, Template_relevance Reaxys, Template_relevance Reaxys_biocatalysis model, leveraging a vast database of chemical reactions to predict feasible synthetic routes.
One-Step Synthesis Focus: Specifically designed for one-step synthesis, it provides concise and direct routes for your target compounds, streamlining the synthesis process.
Accurate Predictions: Utilizing the extensive PISTACHIO, BKMS_METABOLIC, PISTACHIO_RINGBREAKER, REAXYS, REAXYS_BIOCATALYSIS database, our tool offers high-accuracy predictions, reflecting the latest in chemical research and data.
Strategy Settings
Precursor scoring | Relevance Heuristic |
---|---|
Min. plausibility | 0.01 |
Model | Template_relevance |
Template Set | Pistachio/Bkms_metabolic/Pistachio_ringbreaker/Reaxys/Reaxys_biocatalysis |
Top-N result to add to graph | 6 |
Feasible Synthetic Routes
Disclaimer and Information on In-Vitro Research Products
Please be aware that all articles and product information presented on BenchChem are intended solely for informational purposes. The products available for purchase on BenchChem are specifically designed for in-vitro studies, which are conducted outside of living organisms. In-vitro studies, derived from the Latin term "in glass," involve experiments performed in controlled laboratory settings using cells or tissues. It is important to note that these products are not categorized as medicines or drugs, and they have not received approval from the FDA for the prevention, treatment, or cure of any medical condition, ailment, or disease. We must emphasize that any form of bodily introduction of these products into humans or animals is strictly prohibited by law. It is essential to adhere to these guidelines to ensure compliance with legal and ethical standards in research and experimentation.