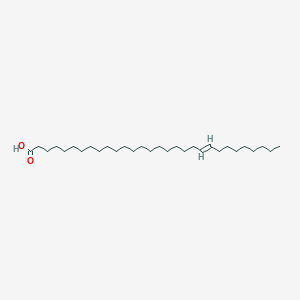
Lumequic acid
- Click on QUICK INQUIRY to receive a quote from our team of experts.
- With the quality product at a COMPETITIVE price, you can focus more on your research.
Overview
Description
Preparation Methods
Synthetic Routes and Reaction Conditions: Lumequic acid can be synthesized through the elongation of shorter fatty acids using specific enzymes or chemical catalysts. The process typically involves the addition of carbon units to the fatty acid chain, resulting in the formation of a very long-chain fatty acid .
Industrial Production Methods: Industrial production of this compound involves the use of biotechnological methods, such as microbial fermentation, where microorganisms are engineered to produce the desired fatty acid. This method is preferred due to its efficiency and sustainability .
Chemical Reactions Analysis
Types of Reactions: Lumequic acid undergoes various chemical reactions, including:
Oxidation: this compound can be oxidized to form shorter-chain fatty acids and other oxidation products.
Reduction: Reduction of this compound can lead to the formation of saturated fatty acids.
Substitution: Substitution reactions can occur at the carboxyl group, leading to the formation of esters and amides.
Common Reagents and Conditions:
Oxidation: Common oxidizing agents include potassium permanganate and chromium trioxide.
Reduction: Reducing agents such as lithium aluminum hydride and hydrogen gas in the presence of a catalyst are used.
Substitution: Reagents like alcohols and amines are used under acidic or basic conditions.
Major Products Formed:
Oxidation: Shorter-chain fatty acids, aldehydes, and ketones.
Reduction: Saturated fatty acids.
Substitution: Esters and amides.
Scientific Research Applications
Lumequic acid has a wide range of applications in scientific research:
Chemistry: It is used as a model compound to study the properties and reactions of very long-chain fatty acids.
Biology: this compound is studied for its role in cellular processes, such as membrane structure and function.
Medicine: Research is ongoing to explore its potential therapeutic applications, including its role in metabolic disorders.
Industry: this compound is used in the production of biodegradable plastics and other sustainable materials.
Mechanism of Action
The mechanism of action of lumequic acid involves its incorporation into cellular membranes, where it influences membrane fluidity and function. It interacts with various molecular targets, including membrane proteins and enzymes, affecting cellular signaling pathways and metabolic processes .
Comparison with Similar Compounds
Arachidic acid: A saturated very long-chain fatty acid with 20 carbon atoms.
Behenic acid: A saturated very long-chain fatty acid with 22 carbon atoms.
Lignoceric acid: A saturated very long-chain fatty acid with 24 carbon atoms.
Uniqueness of Lumequic Acid: this compound is unique due to its specific chain length and the presence of a double bond, which distinguishes it from other very long-chain fatty acids. This structural uniqueness imparts distinct physical and chemical properties, making it valuable for various applications .
Properties
CAS No. |
67329-09-3 |
---|---|
Molecular Formula |
C30H58O2 |
Molecular Weight |
450.8 g/mol |
IUPAC Name |
(Z)-triacont-21-enoic acid |
InChI |
InChI=1S/C30H58O2/c1-2-3-4-5-6-7-8-9-10-11-12-13-14-15-16-17-18-19-20-21-22-23-24-25-26-27-28-29-30(31)32/h9-10H,2-8,11-29H2,1H3,(H,31,32)/b10-9- |
InChI Key |
QXYCISFUJXCMSU-KTKRTIGZSA-N |
Isomeric SMILES |
CCCCCCCC/C=C\CCCCCCCCCCCCCCCCCCCC(=O)O |
SMILES |
CCCCCCCCC=CCCCCCCCCCCCCCCCCCCCC(=O)O |
Canonical SMILES |
CCCCCCCCC=CCCCCCCCCCCCCCCCCCCCC(=O)O |
Appearance |
Solid powder |
melting_point |
60.8-61.2°C |
physical_description |
Solid |
Purity |
>98% (or refer to the Certificate of Analysis) |
shelf_life |
>3 years if stored properly |
solubility |
Soluble in DMSO |
storage |
Dry, dark and at 0 - 4 C for short term (days to weeks) or -20 C for long term (months to years). |
Synonyms |
Lumequeic acid |
Origin of Product |
United States |
Retrosynthesis Analysis
AI-Powered Synthesis Planning: Our tool employs the Template_relevance Pistachio, Template_relevance Bkms_metabolic, Template_relevance Pistachio_ringbreaker, Template_relevance Reaxys, Template_relevance Reaxys_biocatalysis model, leveraging a vast database of chemical reactions to predict feasible synthetic routes.
One-Step Synthesis Focus: Specifically designed for one-step synthesis, it provides concise and direct routes for your target compounds, streamlining the synthesis process.
Accurate Predictions: Utilizing the extensive PISTACHIO, BKMS_METABOLIC, PISTACHIO_RINGBREAKER, REAXYS, REAXYS_BIOCATALYSIS database, our tool offers high-accuracy predictions, reflecting the latest in chemical research and data.
Strategy Settings
Precursor scoring | Relevance Heuristic |
---|---|
Min. plausibility | 0.01 |
Model | Template_relevance |
Template Set | Pistachio/Bkms_metabolic/Pistachio_ringbreaker/Reaxys/Reaxys_biocatalysis |
Top-N result to add to graph | 6 |
Feasible Synthetic Routes
Disclaimer and Information on In-Vitro Research Products
Please be aware that all articles and product information presented on BenchChem are intended solely for informational purposes. The products available for purchase on BenchChem are specifically designed for in-vitro studies, which are conducted outside of living organisms. In-vitro studies, derived from the Latin term "in glass," involve experiments performed in controlled laboratory settings using cells or tissues. It is important to note that these products are not categorized as medicines or drugs, and they have not received approval from the FDA for the prevention, treatment, or cure of any medical condition, ailment, or disease. We must emphasize that any form of bodily introduction of these products into humans or animals is strictly prohibited by law. It is essential to adhere to these guidelines to ensure compliance with legal and ethical standards in research and experimentation.