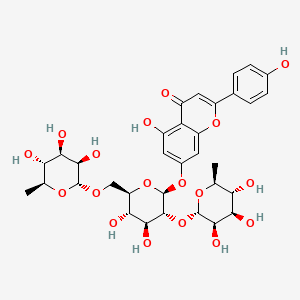
Ligustroflavone
Overview
Description
Ligustroflavone is a bioactive compound derived from the plant Ligustrum lucidum, commonly known as Chinese privet. This compound belongs to the flavonoid class of polyphenolic compounds, which are known for their diverse pharmacological properties. This compound has been studied for its potential therapeutic effects, including anti-inflammatory, antioxidant, and neuroprotective activities .
Mechanism of Action
Target of Action
Ligustroflavone, a major compound found in the fruit of Ligustrum lucidum, has been identified to interact with several primary targets . These include the Calcium-Sensing Receptors (CaSR) , Receptor-Interacting Protein Kinase 1/3 (RIPK1/3) , and Mixed Lineage Kinase Domain-Like (MLKL) . It also shows potential interaction with Tau protein . These targets play crucial roles in various biological processes such as calcium metabolism, necroptosis, and neurodegenerative diseases.
Mode of Action
This compound interacts with its targets to bring about significant changes. It acts as a CaSR antagonist , regulating parathyroid hormone (PTH) levels and improving calcium balance . It also interacts well with the allosteric site of CaSR, increasing PTH release and suppressing extracellular calcium influx . In the context of necroptosis, this compound targets the RIPK1/RIPK3/MLKL pathway, blocking the enhanced interaction between RIPK3 and RIPK1 or MLKL .
Biochemical Pathways
This compound affects several biochemical pathways. It down-regulates the TGF-β/Smad signaling pathway , which plays a crucial role in cellular functions such as cell growth, cell differentiation, apoptosis, and cellular homeostasis. It also impacts the RIPK1/RIPK3/MLKL-mediated necroptosis pathway , which contributes to brain injury after ischemic stroke.
Result of Action
The molecular and cellular effects of this compound’s action are significant. It can transiently increase PTH level, regulate calcium metabolism, and prevent osteoporosis in diabetic mice . It also reduces necroptosis in rat brain after ischemic stroke, protecting the brain from injury . Furthermore, it has been found to have a favorable binding affinity with Tau protein, suggesting potential efficiency towards Alzheimer’s disease .
Biochemical Analysis
Biochemical Properties
Ligustroflavone interacts with various biomolecules, including enzymes and proteins. It has been found to regulate PTH levels and improve calcium balance by acting on CaSR . In a study, this compound interacted well with the allosteric site of CaSR, increased PTH release of primary parathyroid gland cells, and suppressed extracellular calcium influx in HEK-293 cells .
Cellular Effects
This compound has been shown to have significant effects on various types of cells and cellular processes. For instance, it has been found to minimize the impairment of neurological function and block neuronal damage in mice models of ischemic stroke . It also attenuated the upregulation of expression levels of the NLRP1 inflammasome complexes and the inflammatory cytokines TNF-α, IL-18, IL-6, and IL-1β .
Molecular Mechanism
The molecular mechanism of this compound involves its interaction with various biomolecules. It has been found to target RIPK1, RIPK3, and MLKL, which are potential targets of this compound . The interaction between RIPK3 and RIPK1 or MLKL was significantly enhanced, which was blocked in the presence of this compound .
Temporal Effects in Laboratory Settings
In laboratory settings, this compound has been observed to diffuse rapidly and widely into major organs . The level of this compound was highest in the mouse liver, followed by the kidney, spleen, and lung . The overwhelming accumulation in the liver indicated that the liver was responsible for the extensive metabolism .
Dosage Effects in Animal Models
In animal models, the effects of this compound vary with different dosages. For instance, in a study, administration of this compound (30 mg/kg) 15 min before ischemia evidently improved neurological function, reduced infarct volume, and decreased the levels of necroptosis-associated proteins .
Metabolic Pathways
This compound is involved in several metabolic pathways. It has been found to regulate PTH levels and improve calcium balance by acting on CaSR . This suggests that this compound might be involved in the metabolic pathways related to calcium homeostasis.
Transport and Distribution
This compound is transported and distributed within cells and tissues. It diffuses rapidly and widely into major organs . The level of this compound was highest in the mouse liver, followed by the kidney, spleen, and lung .
Preparation Methods
Synthetic Routes and Reaction Conditions: Ligustroflavone can be synthesized through various chemical routes. One common method involves the extraction from the plant Ligustrum lucidum using solvents such as ethanol or methanol. The extract is then subjected to chromatographic techniques to isolate this compound .
Industrial Production Methods: In an industrial setting, this compound is typically produced through large-scale extraction processes. The plant material is harvested, dried, and ground into a fine powder. This powder is then extracted using solvents under controlled conditions to maximize yield. The extract is purified using techniques such as high-performance liquid chromatography (HPLC) to obtain high-purity this compound .
Chemical Reactions Analysis
Types of Reactions: Ligustroflavone undergoes various chemical reactions, including:
Oxidation: this compound can be oxidized to form quinones and other oxidation products.
Reduction: Reduction reactions can convert this compound into its corresponding dihydroflavonoid.
Substitution: this compound can undergo substitution reactions, particularly at the hydroxyl groups, to form various derivatives.
Common Reagents and Conditions:
Oxidation: Common oxidizing agents include hydrogen peroxide and potassium permanganate.
Reduction: Reducing agents such as sodium borohydride are used.
Substitution: Reagents like acyl chlorides and alkyl halides are used for substitution reactions.
Major Products:
Oxidation: Quinones and other oxidized derivatives.
Reduction: Dihydroflavonoid derivatives.
Substitution: Various substituted flavonoid derivatives.
Scientific Research Applications
Chemistry: Used as a reference compound in analytical chemistry for the development of chromatographic methods.
Biology: Studied for its effects on cellular processes, including apoptosis and cell proliferation.
Medicine: Investigated for its neuroprotective effects in models of ischemic stroke and its potential to treat diabetic osteoporosis by acting on calcium-sensing receptors
Industry: Used in the development of nutraceuticals and functional foods due to its antioxidant properties.
Comparison with Similar Compounds
Ligustroflavone is unique among flavonoids due to its specific molecular targets and pathways. Similar compounds include:
Quercetin: Another flavonoid with antioxidant and anti-inflammatory properties.
Kaempferol: Known for its anticancer and cardioprotective effects.
Apigenin: Exhibits anti-inflammatory and anticancer activities
This compound stands out due to its specific action on calcium-sensing receptors and its neuroprotective effects in ischemic stroke models .
Biological Activity
Ligustroflavone (LIG) is a bioactive compound derived from Ligustrum lucidum, known for its diverse pharmacological effects. This article delves into the biological activities of this compound, focusing on its neuroprotective, anti-inflammatory, and hepatoprotective properties, supported by recent research findings.
Neuroprotective Effects
This compound has demonstrated significant neuroprotective activity, particularly in models of ischemic stroke. A study using a rat model of middle cerebral artery occlusion (MCAO) found that this compound administration (30 mg/kg) prior to ischemia improved neurological function and reduced infarct volume. This effect was associated with decreased levels of necroptosis-associated proteins, specifically RIPK1, RIPK3, and MLKL, which are implicated in neuronal cell death following ischemic injury .
Key Findings:
- Improvement in Neurological Function: Administration of this compound led to better scores on neurological assessments post-stroke.
- Reduction in Infarct Volume: Significant decrease in brain tissue damage was observed.
- Mechanism of Action: this compound appears to inhibit necroptosis by targeting RIPK pathways.
Anti-inflammatory Properties
This compound also exhibits notable anti-inflammatory effects. Research indicates that it can inhibit the activation of the NLRP1 inflammasome, which plays a crucial role in inflammatory responses during ischemic injury. In MCAO models, this compound treatment significantly reduced the expression levels of pro-inflammatory cytokines such as IL-1β, IL-18, IL-6, and TNF-α .
Table 1: Inflammatory Cytokine Levels in MCAO Models
Cytokine | MCAO Group (pg/mL) | MCAO + LIG Group (pg/mL) |
---|---|---|
IL-1β | 250 ± 20 | 100 ± 15 |
IL-18 | 300 ± 25 | 120 ± 20 |
IL-6 | 400 ± 30 | 150 ± 25 |
TNF-α | 350 ± 35 | 130 ± 20 |
Hepatoprotective Activity
This compound has been studied for its hepatoprotective effects against liver fibrosis induced by carbon tetrachloride (CCl4). In a mouse model, this compound administration resulted in a reduction of liver fibrosis markers and improved liver function parameters. Histological analysis confirmed decreased collagen deposition and inflammation in treated animals compared to controls .
Table 2: Liver Function Parameters
Parameter | CCl4 Group | CCl4 + LIG Group |
---|---|---|
ALT (U/L) | 150 ± 10 | 80 ± 5 |
AST (U/L) | 180 ± 15 | 90 ± 10 |
Total Bilirubin (mg/dL) | 2.5 ± 0.5 | 1.0 ± 0.2 |
Pharmacokinetics and Tissue Distribution
Pharmacokinetic studies reveal that this compound is rapidly distributed across major organs following administration. It shows the highest concentration in the liver, followed by the kidney and spleen. Notably, its concentration in the brain remains low, suggesting limited ability to cross the blood-brain barrier effectively . This distribution pattern indicates that while this compound may exert significant effects on liver and kidney health, its neuroprotective mechanisms may be more complex due to its limited central nervous system penetration.
Table 3: Tissue Distribution of this compound
Tissue | Concentration (ng/g) |
---|---|
Liver | 5000 |
Kidney | 2000 |
Spleen | 1500 |
Lung | 1000 |
Brain | 100 |
Properties
IUPAC Name |
7-[(2S,3R,4S,5S,6R)-4,5-dihydroxy-3-[(2R,3R,4R,5R,6S)-3,4,5-trihydroxy-6-methyloxan-2-yl]oxy-6-[[(2R,3R,4R,5R,6S)-3,4,5-trihydroxy-6-methyloxan-2-yl]oxymethyl]oxan-2-yl]oxy-5-hydroxy-2-(4-hydroxyphenyl)chromen-4-one | |
---|---|---|
Source | PubChem | |
URL | https://pubchem.ncbi.nlm.nih.gov | |
Description | Data deposited in or computed by PubChem | |
InChI |
InChI=1S/C33H40O18/c1-11-22(37)25(40)28(43)31(46-11)45-10-20-24(39)27(42)30(51-32-29(44)26(41)23(38)12(2)47-32)33(50-20)48-15-7-16(35)21-17(36)9-18(49-19(21)8-15)13-3-5-14(34)6-4-13/h3-9,11-12,20,22-35,37-44H,10H2,1-2H3/t11-,12-,20+,22-,23-,24+,25+,26+,27-,28+,29+,30+,31+,32-,33+/m0/s1 | |
Source | PubChem | |
URL | https://pubchem.ncbi.nlm.nih.gov | |
Description | Data deposited in or computed by PubChem | |
InChI Key |
NULBHTHMVOCGOE-ZBCCAYPVSA-N | |
Source | PubChem | |
URL | https://pubchem.ncbi.nlm.nih.gov | |
Description | Data deposited in or computed by PubChem | |
Canonical SMILES |
CC1C(C(C(C(O1)OCC2C(C(C(C(O2)OC3=CC(=C4C(=C3)OC(=CC4=O)C5=CC=C(C=C5)O)O)OC6C(C(C(C(O6)C)O)O)O)O)O)O)O)O | |
Source | PubChem | |
URL | https://pubchem.ncbi.nlm.nih.gov | |
Description | Data deposited in or computed by PubChem | |
Isomeric SMILES |
C[C@H]1[C@@H]([C@H]([C@H]([C@@H](O1)OC[C@@H]2[C@H]([C@@H]([C@H]([C@@H](O2)OC3=CC(=C4C(=C3)OC(=CC4=O)C5=CC=C(C=C5)O)O)O[C@H]6[C@@H]([C@@H]([C@H]([C@@H](O6)C)O)O)O)O)O)O)O)O | |
Source | PubChem | |
URL | https://pubchem.ncbi.nlm.nih.gov | |
Description | Data deposited in or computed by PubChem | |
Molecular Formula |
C33H40O18 | |
Source | PubChem | |
URL | https://pubchem.ncbi.nlm.nih.gov | |
Description | Data deposited in or computed by PubChem | |
Molecular Weight |
724.7 g/mol | |
Source | PubChem | |
URL | https://pubchem.ncbi.nlm.nih.gov | |
Description | Data deposited in or computed by PubChem | |
CAS No. |
260413-62-5 | |
Record name | Nuezhenoside | |
Source | ChemIDplus | |
URL | https://pubchem.ncbi.nlm.nih.gov/substance/?source=chemidplus&sourceid=0260413625 | |
Description | ChemIDplus is a free, web search system that provides access to the structure and nomenclature authority files used for the identification of chemical substances cited in National Library of Medicine (NLM) databases, including the TOXNET system. | |
Retrosynthesis Analysis
AI-Powered Synthesis Planning: Our tool employs the Template_relevance Pistachio, Template_relevance Bkms_metabolic, Template_relevance Pistachio_ringbreaker, Template_relevance Reaxys, Template_relevance Reaxys_biocatalysis model, leveraging a vast database of chemical reactions to predict feasible synthetic routes.
One-Step Synthesis Focus: Specifically designed for one-step synthesis, it provides concise and direct routes for your target compounds, streamlining the synthesis process.
Accurate Predictions: Utilizing the extensive PISTACHIO, BKMS_METABOLIC, PISTACHIO_RINGBREAKER, REAXYS, REAXYS_BIOCATALYSIS database, our tool offers high-accuracy predictions, reflecting the latest in chemical research and data.
Strategy Settings
Precursor scoring | Relevance Heuristic |
---|---|
Min. plausibility | 0.01 |
Model | Template_relevance |
Template Set | Pistachio/Bkms_metabolic/Pistachio_ringbreaker/Reaxys/Reaxys_biocatalysis |
Top-N result to add to graph | 6 |
Feasible Synthetic Routes
Disclaimer and Information on In-Vitro Research Products
Please be aware that all articles and product information presented on BenchChem are intended solely for informational purposes. The products available for purchase on BenchChem are specifically designed for in-vitro studies, which are conducted outside of living organisms. In-vitro studies, derived from the Latin term "in glass," involve experiments performed in controlled laboratory settings using cells or tissues. It is important to note that these products are not categorized as medicines or drugs, and they have not received approval from the FDA for the prevention, treatment, or cure of any medical condition, ailment, or disease. We must emphasize that any form of bodily introduction of these products into humans or animals is strictly prohibited by law. It is essential to adhere to these guidelines to ensure compliance with legal and ethical standards in research and experimentation.