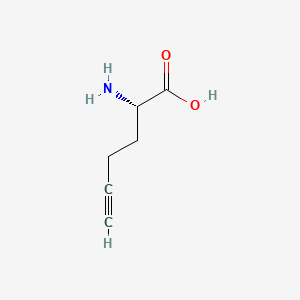
L-homopropargylglycine
Overview
Description
L-homopropargylglycine is an organic compound that belongs to the class of amino acids It features a unique structure with an amino group attached to the second carbon of a hex-5-ynoic acid chain
Preparation Methods
Synthetic Routes and Reaction Conditions
The synthesis of L-homopropargylglycine can be achieved through several methods. One common approach involves the use of starting materials such as hex-5-ynoic acid and appropriate amination reagents. The reaction typically requires specific conditions, including controlled temperature and pH, to ensure the desired stereochemistry is achieved.
Industrial Production Methods
Industrial production of this compound may involve large-scale synthesis using optimized reaction conditions to maximize yield and purity. This often includes the use of catalysts and continuous flow reactors to enhance the efficiency of the process.
Chemical Reactions Analysis
Types of Reactions
L-homopropargylglycine undergoes various chemical reactions, including:
Oxidation: The compound can be oxidized to form corresponding oxo derivatives.
Reduction: Reduction reactions can convert the alkyne group to an alkene or alkane.
Substitution: The amino group can participate in substitution reactions, leading to the formation of derivatives.
Common Reagents and Conditions
Oxidation: Common oxidizing agents include potassium permanganate and ozone.
Reduction: Hydrogenation using palladium on carbon as a catalyst is a typical method.
Substitution: Reagents such as alkyl halides and acyl chlorides are used under basic or acidic conditions.
Major Products Formed
The major products formed from these reactions include various derivatives of this compound, such as oxo derivatives, alkenes, alkanes, and substituted amino acids.
Scientific Research Applications
L-homopropargylglycine has several scientific research applications:
Chemistry: It is used as a building block for the synthesis of more complex molecules.
Biology: The compound is studied for its potential role in metabolic pathways and enzyme interactions.
Medicine: Research is ongoing to explore its potential therapeutic applications, including as a precursor for drug development.
Industry: It is used in the production of specialty chemicals and materials.
Mechanism of Action
The mechanism of action of L-homopropargylglycine involves its interaction with specific molecular targets, such as enzymes and receptors. The compound can modulate biochemical pathways by acting as a substrate or inhibitor, influencing various physiological processes.
Comparison with Similar Compounds
Similar Compounds
(2S)-2-Aminohexanoic acid: Similar structure but lacks the alkyne group.
(2S)-2-Aminopent-4-ynoic acid: Shorter carbon chain with similar functional groups.
(2S)-2-Aminohept-6-ynoic acid: Longer carbon chain with similar functional groups.
Uniqueness
L-homopropargylglycine is unique due to its specific combination of an amino group and an alkyne group on a six-carbon chain. This structure imparts distinct chemical properties and reactivity, making it valuable for specialized applications in research and industry.
Properties
IUPAC Name |
(2S)-2-aminohex-5-ynoic acid | |
---|---|---|
Source | PubChem | |
URL | https://pubchem.ncbi.nlm.nih.gov | |
Description | Data deposited in or computed by PubChem | |
InChI |
InChI=1S/C6H9NO2/c1-2-3-4-5(7)6(8)9/h1,5H,3-4,7H2,(H,8,9)/t5-/m0/s1 | |
Source | PubChem | |
URL | https://pubchem.ncbi.nlm.nih.gov | |
Description | Data deposited in or computed by PubChem | |
InChI Key |
SCGJGNWMYSYORS-YFKPBYRVSA-N | |
Source | PubChem | |
URL | https://pubchem.ncbi.nlm.nih.gov | |
Description | Data deposited in or computed by PubChem | |
Canonical SMILES |
C#CCCC(C(=O)O)N | |
Source | PubChem | |
URL | https://pubchem.ncbi.nlm.nih.gov | |
Description | Data deposited in or computed by PubChem | |
Isomeric SMILES |
C#CCC[C@@H](C(=O)O)N | |
Source | PubChem | |
URL | https://pubchem.ncbi.nlm.nih.gov | |
Description | Data deposited in or computed by PubChem | |
Molecular Formula |
C6H9NO2 | |
Source | PubChem | |
URL | https://pubchem.ncbi.nlm.nih.gov | |
Description | Data deposited in or computed by PubChem | |
Molecular Weight |
127.14 g/mol | |
Source | PubChem | |
URL | https://pubchem.ncbi.nlm.nih.gov | |
Description | Data deposited in or computed by PubChem | |
Retrosynthesis Analysis
AI-Powered Synthesis Planning: Our tool employs the Template_relevance Pistachio, Template_relevance Bkms_metabolic, Template_relevance Pistachio_ringbreaker, Template_relevance Reaxys, Template_relevance Reaxys_biocatalysis model, leveraging a vast database of chemical reactions to predict feasible synthetic routes.
One-Step Synthesis Focus: Specifically designed for one-step synthesis, it provides concise and direct routes for your target compounds, streamlining the synthesis process.
Accurate Predictions: Utilizing the extensive PISTACHIO, BKMS_METABOLIC, PISTACHIO_RINGBREAKER, REAXYS, REAXYS_BIOCATALYSIS database, our tool offers high-accuracy predictions, reflecting the latest in chemical research and data.
Strategy Settings
Precursor scoring | Relevance Heuristic |
---|---|
Min. plausibility | 0.01 |
Model | Template_relevance |
Template Set | Pistachio/Bkms_metabolic/Pistachio_ringbreaker/Reaxys/Reaxys_biocatalysis |
Top-N result to add to graph | 6 |
Feasible Synthetic Routes
Disclaimer and Information on In-Vitro Research Products
Please be aware that all articles and product information presented on BenchChem are intended solely for informational purposes. The products available for purchase on BenchChem are specifically designed for in-vitro studies, which are conducted outside of living organisms. In-vitro studies, derived from the Latin term "in glass," involve experiments performed in controlled laboratory settings using cells or tissues. It is important to note that these products are not categorized as medicines or drugs, and they have not received approval from the FDA for the prevention, treatment, or cure of any medical condition, ailment, or disease. We must emphasize that any form of bodily introduction of these products into humans or animals is strictly prohibited by law. It is essential to adhere to these guidelines to ensure compliance with legal and ethical standards in research and experimentation.