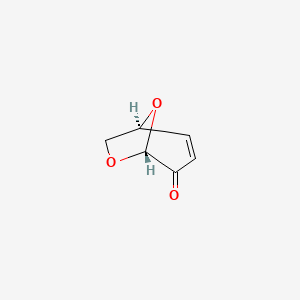
Levoglucosenone
Overview
Description
Levoglucosenone is an organic compound with the formula C₆H₆O₃. It is a pale yellow liquid and an unsaturated bicyclic ketone-diether formed from levoglucosan by the loss of two molecules of water. This compound is of significant interest due to its potential as a biofuel and biofeedstock .
Mechanism of Action
Target of Action
Levoglucosenone (LGO) is a bio-privileged molecule that can be produced on scale from waste biomass . It is a chiral building block that has been converted via well-established chemical processes into previously difficult-to-synthesize building blocks . The primary targets of LGO are the biochemical pathways that these building blocks are involved in.
Mode of Action
The mode of action of LGO involves its interaction with these biochemical pathways. The α,β-unsaturated ketone and masked aldehyde functionalities in this compound open the way to a wide range of synthetic strategies . These include 1,2-addition, α-substitution, cycloaddition/cyclization, and conjugate addition reactions .
Biochemical Pathways
LGO affects several biochemical pathways. It can be converted to different high added-value chemicals such as this compound, 5-hydroxymethylfurfural, and styrene directly or through a glucose intermediate via chemical, catalytic, and biochemical processes . The downstream effects of these pathways result in the production of a wide range of bio-based fine chemicals and polymers, as well as green solvents .
Result of Action
The result of LGO’s action is the production of a multitude of high-added-value compounds. Furthermore, structural resemblance to nature-derived or -inspired target molecules reduces the synthetic steps required compared to their petrochemical counterparts . LGO is an excellent starting material for the synthesis of biologically active compounds, including those which have anti-cancer, anti-microbial, or anti-inflammatory activity .
Action Environment
The action of LGO is influenced by environmental factors. For instance, the use of polar, aprotic solvents such as THF, γ-valerolactone, and sulfolane has been found to improve pyrolytic yields, as the solvents cause swelling of the cellulose and inhibit repolymerisation back to levoglucosan . These solvents also promote catalytic dehydration of levoglucosan to this compound . Therefore, the environment in which LGO is produced and used can significantly influence its action, efficacy, and stability.
Biochemical Analysis
Biochemical Properties
Levoglucosenone plays a crucial role in various biochemical reactions. It interacts with several enzymes, proteins, and other biomolecules. For instance, in the conversion process to 5-hydroxymethylfurfural, this compound interacts with oxalic acid as a catalyst, significantly enhancing its conversion . The nature of these interactions often involves catalytic processes that increase the yield and selectivity of the desired products.
Cellular Effects
This compound influences various types of cells and cellular processes. It has been observed to affect cell signaling pathways, gene expression, and cellular metabolism. For example, in the production of bio-oils, this compound’s interaction with cellulose-derived compounds can lead to changes in cellular metabolism, promoting the formation of high-value chemicals . These effects are crucial for understanding how this compound can be utilized in biotechnological applications.
Molecular Mechanism
The mechanism of action of this compound at the molecular level involves several binding interactions with biomolecules. It can act as an enzyme inhibitor or activator, depending on the context of its use. For instance, in the catalytic conversion process, this compound binds with specific catalysts, facilitating the transformation into other valuable chemicals . These interactions often result in changes in gene expression, further influencing the biochemical pathways involved.
Temporal Effects in Laboratory Settings
In laboratory settings, the effects of this compound change over time. Its stability and degradation are critical factors that influence its long-term effects on cellular function. Studies have shown that this compound remains stable under specific conditions, allowing for prolonged biochemical reactions . Its degradation products can also impact cellular processes, necessitating careful monitoring in experimental setups.
Dosage Effects in Animal Models
The effects of this compound vary with different dosages in animal models. At lower doses, it may exhibit beneficial effects, such as promoting the production of bio-renewable chemicals. At higher doses, this compound can exhibit toxic or adverse effects . Understanding these threshold effects is essential for optimizing its use in various applications.
Metabolic Pathways
This compound is involved in several metabolic pathways, interacting with various enzymes and cofactors. For example, in the production of 5-hydroxymethylfurfural, this compound undergoes catalytic conversion, involving multiple enzymatic steps . These interactions can affect metabolic flux and metabolite levels, highlighting the importance of this compound in metabolic engineering.
Transport and Distribution
Within cells and tissues, this compound is transported and distributed through specific transporters and binding proteins. These interactions influence its localization and accumulation, affecting its overall activity and function . Understanding these transport mechanisms is crucial for optimizing the use of this compound in biotechnological applications.
Subcellular Localization
This compound’s subcellular localization plays a significant role in its activity and function. It may be directed to specific compartments or organelles through targeting signals or post-translational modifications . These localization patterns can influence its interactions with other biomolecules, further affecting its biochemical properties and cellular effects.
Preparation Methods
Synthetic Routes and Reaction Conditions: Levoglucosenone is primarily obtained via the pyrolysis of carbohydrates, particularly cellulose. The process involves heating cellulose above 170°C with sulfuric acid and various additives . The use of polar, aprotic solvents such as tetrahydrofuran (THF), γ-valerolactone, and sulfolane has been found to improve pyrolytic yields by causing swelling of the cellulose and inhibiting repolymerization back to levoglucosan .
Industrial Production Methods: Industrial production of this compound involves the fast pyrolysis of acid-impregnated cellulose. The impregnation of acid reduces the activation energy and lowers the reaction temperature, significantly increasing the yield of this compound . Additionally, microwave irradiation of microcrystalline cellulose can also be used to produce this compound .
Chemical Reactions Analysis
Types of Reactions: Levoglucosenone undergoes various chemical reactions, including:
Oxidation: Allylic oxidation using manganese dioxide (MnO₂).
Reduction: Catalytic hydrogenation to produce dihydrothis compound (Cyrene).
Substitution: Reactions with diazo compounds to form pyrazolines.
Cycloaddition: Diels-Alder reactions with dienes.
Common Reagents and Conditions:
Oxidation: MnO₂ as the oxidizing agent.
Reduction: Palladium on alumina (Pd/Al₂O₃) catalyst at low temperatures (40°C) for selective hydrogenation.
Substitution: Methyl diazoacetate for reactions with diazo compounds.
Cycloaddition: Ethyl vinyl ether and 2,3-dimethylbutadiene for Diels-Alder reactions.
Major Products:
Oxidation: Allylic alcohols.
Reduction: Dihydrothis compound (Cyrene).
Substitution: Pyrazolines.
Cycloaddition: Bicyclic enones and vinyl ethers.
Scientific Research Applications
Levoglucosenone has a wide range of applications in scientific research:
Chemistry: Used as a precursor for the synthesis of various fine chemicals and polymers.
Biology: Investigated for its potential as a bio-renewable platform for the production of commodity chemicals.
Medicine: Explored for its use in drug synthesis and as a chiral building block.
Industry: Utilized in the production of bio-based solvents and as a feedstock for biofuel production.
Comparison with Similar Compounds
Levoglucosan: An anhydrous sugar formed during the pyrolysis of cellulose.
Dihydrolevoglucosenone (Cyrene): A biomass-derived solvent produced by the hydrogenation of this compound.
5-Hydroxymethylfurfural (HMF): A compound formed from the dehydration of sugars and used as a platform chemical.
Uniqueness of this compound: this compound stands out due to its high reactivity and versatility in chemical synthesis. Its ability to undergo a wide range of reactions, including oxidation, reduction, substitution, and cycloaddition, makes it a valuable compound in various fields of research and industry .
Properties
IUPAC Name |
(1S,5R)-6,8-dioxabicyclo[3.2.1]oct-2-en-4-one | |
---|---|---|
Source | PubChem | |
URL | https://pubchem.ncbi.nlm.nih.gov | |
Description | Data deposited in or computed by PubChem | |
InChI |
InChI=1S/C6H6O3/c7-5-2-1-4-3-8-6(5)9-4/h1-2,4,6H,3H2/t4-,6+/m0/s1 | |
Source | PubChem | |
URL | https://pubchem.ncbi.nlm.nih.gov | |
Description | Data deposited in or computed by PubChem | |
InChI Key |
HITOXZPZGPXYHY-UJURSFKZSA-N | |
Source | PubChem | |
URL | https://pubchem.ncbi.nlm.nih.gov | |
Description | Data deposited in or computed by PubChem | |
Canonical SMILES |
C1C2C=CC(=O)C(O1)O2 | |
Source | PubChem | |
URL | https://pubchem.ncbi.nlm.nih.gov | |
Description | Data deposited in or computed by PubChem | |
Isomeric SMILES |
C1[C@@H]2C=CC(=O)[C@H](O1)O2 | |
Source | PubChem | |
URL | https://pubchem.ncbi.nlm.nih.gov | |
Description | Data deposited in or computed by PubChem | |
Molecular Formula |
C6H6O3 | |
Source | PubChem | |
URL | https://pubchem.ncbi.nlm.nih.gov | |
Description | Data deposited in or computed by PubChem | |
Molecular Weight |
126.11 g/mol | |
Source | PubChem | |
URL | https://pubchem.ncbi.nlm.nih.gov | |
Description | Data deposited in or computed by PubChem | |
CAS No. |
37112-31-5 | |
Record name | Levoglucosenone | |
Source | CAS Common Chemistry | |
URL | https://commonchemistry.cas.org/detail?cas_rn=37112-31-5 | |
Description | CAS Common Chemistry is an open community resource for accessing chemical information. Nearly 500,000 chemical substances from CAS REGISTRY cover areas of community interest, including common and frequently regulated chemicals, and those relevant to high school and undergraduate chemistry classes. This chemical information, curated by our expert scientists, is provided in alignment with our mission as a division of the American Chemical Society. | |
Explanation | The data from CAS Common Chemistry is provided under a CC-BY-NC 4.0 license, unless otherwise stated. | |
Record name | 1,6-Anhydro-3,4-dideoxyhex-3-enopyran-2-ulose | |
Source | ChemIDplus | |
URL | https://pubchem.ncbi.nlm.nih.gov/substance/?source=chemidplus&sourceid=0037112315 | |
Description | ChemIDplus is a free, web search system that provides access to the structure and nomenclature authority files used for the identification of chemical substances cited in National Library of Medicine (NLM) databases, including the TOXNET system. | |
Record name | LEVOGLUCOSENONE | |
Source | FDA Global Substance Registration System (GSRS) | |
URL | https://gsrs.ncats.nih.gov/ginas/app/beta/substances/B7N23SN1LB | |
Description | The FDA Global Substance Registration System (GSRS) enables the efficient and accurate exchange of information on what substances are in regulated products. Instead of relying on names, which vary across regulatory domains, countries, and regions, the GSRS knowledge base makes it possible for substances to be defined by standardized, scientific descriptions. | |
Explanation | Unless otherwise noted, the contents of the FDA website (www.fda.gov), both text and graphics, are not copyrighted. They are in the public domain and may be republished, reprinted and otherwise used freely by anyone without the need to obtain permission from FDA. Credit to the U.S. Food and Drug Administration as the source is appreciated but not required. | |
Retrosynthesis Analysis
AI-Powered Synthesis Planning: Our tool employs the Template_relevance Pistachio, Template_relevance Bkms_metabolic, Template_relevance Pistachio_ringbreaker, Template_relevance Reaxys, Template_relevance Reaxys_biocatalysis model, leveraging a vast database of chemical reactions to predict feasible synthetic routes.
One-Step Synthesis Focus: Specifically designed for one-step synthesis, it provides concise and direct routes for your target compounds, streamlining the synthesis process.
Accurate Predictions: Utilizing the extensive PISTACHIO, BKMS_METABOLIC, PISTACHIO_RINGBREAKER, REAXYS, REAXYS_BIOCATALYSIS database, our tool offers high-accuracy predictions, reflecting the latest in chemical research and data.
Strategy Settings
Precursor scoring | Relevance Heuristic |
---|---|
Min. plausibility | 0.01 |
Model | Template_relevance |
Template Set | Pistachio/Bkms_metabolic/Pistachio_ringbreaker/Reaxys/Reaxys_biocatalysis |
Top-N result to add to graph | 6 |
Feasible Synthetic Routes
Q1: What is the molecular formula and weight of levoglucosenone?
A1: this compound has the molecular formula C6H6O3 and a molecular weight of 126.11 g/mol. []
Q2: Is there any spectroscopic data available for this compound?
A2: Yes, researchers frequently utilize techniques like Nuclear Magnetic Resonance (NMR) spectroscopy (both 1H and 13C) and X-ray crystallography to elucidate the structures of this compound derivatives and confirm stereochemical assignments. [, , ]
Q3: Is this compound stable in aqueous solutions?
A3: this compound exhibits degradation in aqueous solutions, with the degradation rate being influenced by factors such as solvent, pH, and temperature. It's crucial to analyze both this compound standard solutions and samples promptly after preparation. A primary degradation product identified is furfural. []
Q4: How is this compound produced from cellulose?
A4: this compound can be produced through the catalytic fast pyrolysis of cellulose. Various catalysts have been investigated, including sulfated titania [] and phosphoric acid. [, , ] The yield of this compound is influenced by factors such as pyrolysis temperature, catalyst type, and cellulose-to-catalyst ratio.
Q5: Can ionic liquids be used to improve the yield of this compound during cellulose pyrolysis?
A5: Yes, specific ionic liquids, particularly those with sulfonate anions, have demonstrated significant catalytic activity in cellulose pyrolysis, leading to enhanced yields of this compound and allowing for the recovery and reuse of the ionic liquid. [, ]
Q6: Are there alternative methods for producing this compound from cellulose besides pyrolysis?
A6: Yes, plasma electrolysis has emerged as a promising low-energy method for producing this compound from cellulose in polar aprotic solvents. This method, employing high-voltage alternating current, enables complete cellulose liquefaction without external heating, leading to high this compound yields with reduced energy consumption compared to conventional methods. [, ]
Q7: What are some notable applications of this compound in organic synthesis?
A7: this compound serves as a valuable chiral starting material for synthesizing various compounds, including: * Carbohydrate-based molecules: It has been employed in the synthesis of unnatural (1→6)-polysaccharides, showcasing potential in materials science. [] * Bio-based solvents: Derivatives like Cyrene and its ketal variants (dioxolane Cygnet, dioxane Cygnet, and dioxepane Cygnet) have been synthesized and explored as green alternatives for polyester synthesis and processing. [] * Chiral auxiliaries: A new family of chiral auxiliaries derived from this compound has shown promise in facilitating diastereoselective Diels-Alder reactions, enabling the synthesis of complex molecules. [] * Thiosemicarbazones: this compound-derived thiosemicarbazones have demonstrated promising antimycobacterial activity, highlighting their potential as leads for developing novel antituberculosis agents. []
Q8: Can this compound be used to synthesize other important building blocks?
A8: Absolutely! this compound can be transformed into isothis compound, its regioisomer, which also holds potential as a chiral building block. [] This conversion can be achieved using Wharton rearrangement chemistry or through lipase-mediated kinetic resolution. [, ]
Q9: How does the structure of this compound contribute to its synthetic utility?
A9: this compound's rigid bicyclic framework, with its differentiated enone faces due to the 1,6-anhydro bridge, enables high levels of regio- and stereocontrol in various reactions, including Diels-Alder reactions [, ], 1,3-dipolar cycloadditions [], and Michael additions. [, , ]
Q10: What types of reactions can be performed with this compound?
A10: this compound is highly versatile, readily undergoing a range of reactions including: * Michael additions: Both base-catalyzed and electrochemically promoted Michael additions have been successfully applied to this compound, allowing the introduction of various functionalities. [, ] * Diels-Alder reactions: this compound acts as a potent dienophile in Diels-Alder reactions, offering a route to complex polycyclic structures. [, ] * Aziridination: Stereoselective aziridination of this compound is achievable, leading to the formation of unique 6,8-dioxabicyclo[3.2.1]oct-3-enes, valuable as sp3-rich chiral scaffolds in drug discovery. [] * Ring-opening reactions: The anhydro bridge in this compound can undergo ring-opening, providing opportunities for further structural elaboration. []
Q11: Has this compound been investigated for any biological activity?
A11: Yes, research has explored the biological activity of this compound derivatives. For instance, medium and large lactones synthesized from this compound have undergone in vitro antifungal, antimicrobial, and antitumor activity screening. [] Some derivatives have displayed moderate fungistatic activity against plant pathogens and weak cytotoxic properties against cancer cell lines. []
Q12: Are there any environmental considerations related to this compound?
A12: While this compound itself is considered a valuable renewable building block, its production from biomass, particularly the pyrolysis of treated wood, requires careful consideration regarding the potential release of harmful byproducts. For example, pyrolysis of chromated copper arsenate (CCA)-treated wood, while a potential source of this compound, necessitates careful management due to the presence of toxic metals. []
Disclaimer and Information on In-Vitro Research Products
Please be aware that all articles and product information presented on BenchChem are intended solely for informational purposes. The products available for purchase on BenchChem are specifically designed for in-vitro studies, which are conducted outside of living organisms. In-vitro studies, derived from the Latin term "in glass," involve experiments performed in controlled laboratory settings using cells or tissues. It is important to note that these products are not categorized as medicines or drugs, and they have not received approval from the FDA for the prevention, treatment, or cure of any medical condition, ailment, or disease. We must emphasize that any form of bodily introduction of these products into humans or animals is strictly prohibited by law. It is essential to adhere to these guidelines to ensure compliance with legal and ethical standards in research and experimentation.