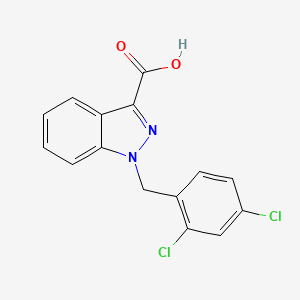
Lonidamine
Overview
Description
Lonidamine is a derivative of indazole-3-carboxylic acid, known for its ability to inhibit aerobic glycolysis in cancer cells. It was first introduced in 1979 as an anti-spermatogenic agent but later found to have antitumor activity by interfering with the energy metabolism of cancer cells .
Mechanism of Action
Target of Action
Lonidamine (LND) primarily targets the hexokinase-1 (HK1) enzyme and the cystic fibrosis transmembrane conductance regulator (CFTR) . HK1 is an enzyme that catalyzes the first step in glycolysis, the process by which glucose is broken down to produce energy . CFTR is a protein that regulates the movement of ions across cell membranes .
Mode of Action
LND is an orally administered small molecule that inhibits glycolysis by inactivating hexokinase . This inhibition of hexokinase is well established . In addition, there is evidence that LND may increase programmed cell death . This stems from the observation that mitochondria and mitochondria-bound hexokinase are crucial for the induction of apoptosis .
Pharmacokinetics
After single administration, the plasma kinetics are highly variable, while the dose eliminated in the urine is over 70% in all subjects . This suggests an active but interindividually variable first-pass effect .
Result of Action
LND blocks lung tumor development and brain metastasis by inhibiting mitochondrial bioenergetics, stimulating the formation of reactive oxygen species, oxidizing mitochondrial peroxiredoxin . It also inactivates AKT/mTOR/p70S6K signaling, and induces autophagic cell death in lung cancer cells .
Action Environment
The action of LND is influenced by the pH of the environment. LND is more soluble at alkaline pH, suggesting that LND has specificity to the alkaline intracellular microenvironment of cancer cells, where it will exhibit its greatest effects in combination with other therapeutic agents .
Biochemical Analysis
Biochemical Properties
Lonidamine plays a crucial role in biochemical reactions by inhibiting glycolysis. It primarily interacts with hexokinase, an enzyme that catalyzes the first step in glycolysis by converting glucose to glucose-6-phosphate. This compound inactivates hexokinase, thereby disrupting the glycolytic pathway. Additionally, this compound targets monocarboxylate transporters, mitochondrial pyruvate carriers, respiratory chain complexes I and II, mitochondrial permeability transition pores, and hexokinase II. These interactions collectively inhibit energy production in cancer cells, leading to reduced tumor growth .
Cellular Effects
This compound exerts significant effects on various types of cells and cellular processes. In cancer cells, it inhibits aerobic glycolysis, leading to decreased energy production and cell proliferation. This inhibition affects cell signaling pathways, gene expression, and cellular metabolism. For instance, in human malignant gliomas, this compound has been shown to inhibit lactate production, which is a key indicator of glycolytic activity. This inhibition is more pronounced in higher-grade tumors, suggesting a correlation between this compound’s effects and tumor malignancy .
Molecular Mechanism
The molecular mechanism of this compound involves its interaction with several key biomolecules. By binding to hexokinase, this compound inhibits its activity, thereby blocking the glycolytic pathway. This inhibition reduces the availability of ATP, which is essential for cancer cell survival and proliferation. Additionally, this compound affects mitochondrial function by targeting mitochondrial pyruvate carriers and respiratory chain complexes, leading to impaired oxidative phosphorylation. These combined effects result in decreased energy production and increased apoptosis in cancer cells .
Temporal Effects in Laboratory Settings
In laboratory settings, the effects of this compound have been observed to change over time. This compound exhibits stability under standard laboratory conditions, but its effects on cellular function can vary depending on the duration of exposure. Short-term exposure to this compound leads to immediate inhibition of glycolysis and reduced cell proliferation. Long-term exposure can result in adaptive responses, where cancer cells may develop resistance to this compound’s effects. Additionally, this compound’s degradation products may also influence its long-term efficacy .
Dosage Effects in Animal Models
The effects of this compound vary with different dosages in animal models. At low doses, this compound effectively inhibits glycolysis and reduces tumor growth without causing significant toxicity. At higher doses, this compound can induce toxic effects, such as hepatotoxicity and nephrotoxicity. These adverse effects highlight the importance of optimizing the dosage of this compound to achieve maximum therapeutic benefit while minimizing toxicity. Threshold effects have also been observed, where a minimum effective dose is required to achieve significant anticancer activity .
Metabolic Pathways
This compound is involved in several metabolic pathways, primarily targeting glycolysis. By inhibiting hexokinase, this compound disrupts the conversion of glucose to glucose-6-phosphate, leading to reduced glycolytic flux. This inhibition affects downstream metabolic pathways, including the tricarboxylic acid cycle and oxidative phosphorylation. Additionally, this compound’s interaction with monocarboxylate transporters and mitochondrial pyruvate carriers further impairs energy production in cancer cells .
Transport and Distribution
Within cells and tissues, this compound is transported and distributed through various mechanisms. It interacts with transporters and binding proteins that facilitate its uptake and localization. This compound’s distribution is influenced by its lipophilic nature, allowing it to accumulate in cellular membranes and organelles. This accumulation enhances its inhibitory effects on glycolysis and mitochondrial function. Additionally, this compound’s interaction with monocarboxylate transporters affects its transport across cellular membranes .
Subcellular Localization
This compound’s subcellular localization plays a crucial role in its activity and function. It primarily localizes to mitochondria, where it interacts with mitochondrial pyruvate carriers, respiratory chain complexes, and hexokinase II. This localization is facilitated by targeting signals and post-translational modifications that direct this compound to specific compartments within the cell. The accumulation of this compound in mitochondria enhances its inhibitory effects on energy production and promotes apoptosis in cancer cells .
Preparation Methods
Synthetic Routes and Reaction Conditions
Lonidamine can be synthesized through a multi-step process involving the reaction of 2,4-dichlorobenzyl chloride with indazole-3-carboxylic acid. The reaction typically involves the use of a base such as sodium hydroxide or potassium carbonate in an organic solvent like dimethylformamide (DMF) or dimethyl sulfoxide (DMSO). The reaction is carried out under reflux conditions to yield this compound .
Industrial Production Methods
Industrial production of this compound involves similar synthetic routes but on a larger scale. The process is optimized for higher yields and purity, often involving additional purification steps such as recrystallization or chromatography to ensure the final product meets pharmaceutical standards .
Chemical Reactions Analysis
Types of Reactions
Lonidamine undergoes various chemical reactions, including:
Oxidation: this compound can be oxidized under specific conditions, leading to the formation of different oxidation products.
Reduction: Reduction reactions can modify the functional groups in this compound, potentially altering its biological activity.
Substitution: Substitution reactions, particularly nucleophilic substitutions, can occur at the benzyl chloride moiety
Common Reagents and Conditions
Oxidation: Common oxidizing agents include potassium permanganate and hydrogen peroxide.
Reduction: Reducing agents such as lithium aluminum hydride (LiAlH4) or sodium borohydride (NaBH4) are used.
Substitution: Nucleophiles like amines or thiols can be used in substitution reactions
Major Products
The major products formed from these reactions depend on the specific conditions and reagents used. For example, oxidation can lead to the formation of carboxylic acids or ketones, while reduction can yield alcohols or amines .
Scientific Research Applications
Lonidamine has a wide range of scientific research applications:
Chemistry: Used as a model compound to study the effects of glycolysis inhibition.
Biology: Investigated for its effects on cellular metabolism and energy production.
Medicine: Explored as a potential anticancer agent due to its ability to inhibit glycolysis in cancer cells. .
Industry: Utilized in the development of new drug delivery systems, such as liposomes and polymeric micelles, to improve the bioavailability and targeting of chemotherapeutic agents
Comparison with Similar Compounds
Similar Compounds
Dichloroacetate: Another glycolysis inhibitor that targets pyruvate dehydrogenase kinase.
2-Deoxy-D-glucose: A glucose analog that inhibits glycolysis by competing with glucose for uptake and phosphorylation.
Gamendazole: A derivative of lonidamine being investigated as a potential male contraceptive
Uniqueness
This compound is unique in its dual action on both glycolysis and mitochondrial function, making it a potent inhibitor of cancer cell metabolism. Its ability to enhance the efficacy of other anticancer agents and therapies further distinguishes it from other glycolysis inhibitors .
Biological Activity
Lonidamine (LND) is a compound that has garnered attention for its diverse biological activities, particularly in the context of cancer treatment and metabolic regulation. Originally developed as an anti-spermatogenic agent, LND has been repurposed in recent years for its potential anti-cancer properties. This article delves into the biological activity of this compound, focusing on its mechanisms of action, pharmacodynamics, and clinical implications.
This compound exerts its effects primarily through the inhibition of glycolysis and mitochondrial functions. The following key mechanisms have been identified:
- Inhibition of Glycolysis : LND selectively inhibits hexokinase II (HK-II), an enzyme crucial for glucose metabolism in cancer cells. This inhibition leads to decreased glucose phosphorylation and reduced levels of glycolytic intermediates, ultimately resulting in lower lactate production and cellular acidification .
- Mitochondrial Effects : LND disrupts mitochondrial bioenergetics by inhibiting complexes I and II of the mitochondrial electron transport chain. This inhibition promotes the generation of reactive oxygen species (ROS), which can induce apoptosis in cancer cells .
- Mitochondrial Permeability Transition : LND opens the mitochondrial permeability transition pore, facilitating the release of pro-apoptotic factors such as cytochrome c, which further drives apoptotic signaling pathways .
Pharmacodynamics
The pharmacodynamics of this compound highlight its selective action on tumor cells compared to normal cells. Studies indicate that LND's inhibitory effects on glycolysis are more pronounced in highly undifferentiated tumor cells, suggesting a potential therapeutic window for its application in oncology .
Table 1: Summary of this compound's Mechanisms
Case Studies and Research Findings
- Combination Therapy : Recent studies have explored the use of this compound in combination with other chemotherapeutic agents. For instance, Mito-LND, a mitochondria-targeted derivative of this compound, has shown enhanced efficacy against lung cancer by suppressing tumor growth and metastasis without significant toxicity .
- Anti-Cancer Efficacy : In preclinical models, this compound has demonstrated significant anti-tumor activity across various cancer types. For example, its ability to induce apoptosis through mitochondrial pathways has been validated in glioma and lung cancer cell lines .
- Synergistic Effects : Research indicates that combining this compound with other agents can yield synergistic effects, enhancing overall treatment efficacy. For example, studies have shown that combining LND with arsenic trioxide (ATO) can improve outcomes in certain cancer models by modulating signaling pathways involved in cell survival and apoptosis .
Properties
IUPAC Name |
1-[(2,4-dichlorophenyl)methyl]indazole-3-carboxylic acid | |
---|---|---|
Source | PubChem | |
URL | https://pubchem.ncbi.nlm.nih.gov | |
Description | Data deposited in or computed by PubChem | |
InChI |
InChI=1S/C15H10Cl2N2O2/c16-10-6-5-9(12(17)7-10)8-19-13-4-2-1-3-11(13)14(18-19)15(20)21/h1-7H,8H2,(H,20,21) | |
Source | PubChem | |
URL | https://pubchem.ncbi.nlm.nih.gov | |
Description | Data deposited in or computed by PubChem | |
InChI Key |
WDRYRZXSPDWGEB-UHFFFAOYSA-N | |
Source | PubChem | |
URL | https://pubchem.ncbi.nlm.nih.gov | |
Description | Data deposited in or computed by PubChem | |
Canonical SMILES |
C1=CC=C2C(=C1)C(=NN2CC3=C(C=C(C=C3)Cl)Cl)C(=O)O | |
Source | PubChem | |
URL | https://pubchem.ncbi.nlm.nih.gov | |
Description | Data deposited in or computed by PubChem | |
Molecular Formula |
C15H10Cl2N2O2 | |
Source | PubChem | |
URL | https://pubchem.ncbi.nlm.nih.gov | |
Description | Data deposited in or computed by PubChem | |
DSSTOX Substance ID |
DTXSID5020782 | |
Record name | 1-(2,4-Dichlorobenzyl)-1H-indazole-3-carboxylic acid | |
Source | EPA DSSTox | |
URL | https://comptox.epa.gov/dashboard/DTXSID5020782 | |
Description | DSSTox provides a high quality public chemistry resource for supporting improved predictive toxicology. | |
Molecular Weight |
321.2 g/mol | |
Source | PubChem | |
URL | https://pubchem.ncbi.nlm.nih.gov | |
Description | Data deposited in or computed by PubChem | |
Mechanism of Action |
Lonidamine is an orally administered small molecule that inhibits glycolysis by the inactivation of hexokinase. Hexokinase is an enzyme that catalyzes glucose, the first step in glycolysis. The inhibition of hexokinase by lonidamine is well established. In addition, there is evidence that lonidamine may increase programmed cell death. This stems from the observation that mitochondria and mitochondria-bound hexokinase are crucial for induction of apoptosis; agents that directly effect mitochondria may, therefore, trigger apoptosis. Indeed, in vitro models with lonidamine exhibit the hallmarks of apoptosis, including mitochondrial membrane depolarization, release of cytochrome C, phosphatidylserine externalization, and DNA fragmentation. [PMID: 16986057] | |
Record name | Lonidamine | |
Source | DrugBank | |
URL | https://www.drugbank.ca/drugs/DB06266 | |
Description | The DrugBank database is a unique bioinformatics and cheminformatics resource that combines detailed drug (i.e. chemical, pharmacological and pharmaceutical) data with comprehensive drug target (i.e. sequence, structure, and pathway) information. | |
Explanation | Creative Common's Attribution-NonCommercial 4.0 International License (http://creativecommons.org/licenses/by-nc/4.0/legalcode) | |
CAS No. |
50264-69-2 | |
Record name | Lonidamine | |
Source | CAS Common Chemistry | |
URL | https://commonchemistry.cas.org/detail?cas_rn=50264-69-2 | |
Description | CAS Common Chemistry is an open community resource for accessing chemical information. Nearly 500,000 chemical substances from CAS REGISTRY cover areas of community interest, including common and frequently regulated chemicals, and those relevant to high school and undergraduate chemistry classes. This chemical information, curated by our expert scientists, is provided in alignment with our mission as a division of the American Chemical Society. | |
Explanation | The data from CAS Common Chemistry is provided under a CC-BY-NC 4.0 license, unless otherwise stated. | |
Record name | Lonidamine [INN:BAN] | |
Source | ChemIDplus | |
URL | https://pubchem.ncbi.nlm.nih.gov/substance/?source=chemidplus&sourceid=0050264692 | |
Description | ChemIDplus is a free, web search system that provides access to the structure and nomenclature authority files used for the identification of chemical substances cited in National Library of Medicine (NLM) databases, including the TOXNET system. | |
Record name | Lonidamine | |
Source | DrugBank | |
URL | https://www.drugbank.ca/drugs/DB06266 | |
Description | The DrugBank database is a unique bioinformatics and cheminformatics resource that combines detailed drug (i.e. chemical, pharmacological and pharmaceutical) data with comprehensive drug target (i.e. sequence, structure, and pathway) information. | |
Explanation | Creative Common's Attribution-NonCommercial 4.0 International License (http://creativecommons.org/licenses/by-nc/4.0/legalcode) | |
Record name | lonidamine | |
Source | DTP/NCI | |
URL | https://dtp.cancer.gov/dtpstandard/servlet/dwindex?searchtype=NSC&outputformat=html&searchlist=758419 | |
Description | The NCI Development Therapeutics Program (DTP) provides services and resources to the academic and private-sector research communities worldwide to facilitate the discovery and development of new cancer therapeutic agents. | |
Explanation | Unless otherwise indicated, all text within NCI products is free of copyright and may be reused without our permission. Credit the National Cancer Institute as the source. | |
Record name | lonidamine | |
Source | DTP/NCI | |
URL | https://dtp.cancer.gov/dtpstandard/servlet/dwindex?searchtype=NSC&outputformat=html&searchlist=741419 | |
Description | The NCI Development Therapeutics Program (DTP) provides services and resources to the academic and private-sector research communities worldwide to facilitate the discovery and development of new cancer therapeutic agents. | |
Explanation | Unless otherwise indicated, all text within NCI products is free of copyright and may be reused without our permission. Credit the National Cancer Institute as the source. | |
Record name | 1-(2,4-Dichlorobenzyl)-1H-indazole-3-carboxylic acid | |
Source | EPA DSSTox | |
URL | https://comptox.epa.gov/dashboard/DTXSID5020782 | |
Description | DSSTox provides a high quality public chemistry resource for supporting improved predictive toxicology. | |
Record name | Lonidamine | |
Source | European Chemicals Agency (ECHA) | |
URL | https://echa.europa.eu/substance-information/-/substanceinfo/100.051.356 | |
Description | The European Chemicals Agency (ECHA) is an agency of the European Union which is the driving force among regulatory authorities in implementing the EU's groundbreaking chemicals legislation for the benefit of human health and the environment as well as for innovation and competitiveness. | |
Explanation | Use of the information, documents and data from the ECHA website is subject to the terms and conditions of this Legal Notice, and subject to other binding limitations provided for under applicable law, the information, documents and data made available on the ECHA website may be reproduced, distributed and/or used, totally or in part, for non-commercial purposes provided that ECHA is acknowledged as the source: "Source: European Chemicals Agency, http://echa.europa.eu/". Such acknowledgement must be included in each copy of the material. ECHA permits and encourages organisations and individuals to create links to the ECHA website under the following cumulative conditions: Links can only be made to webpages that provide a link to the Legal Notice page. | |
Record name | LONIDAMINE | |
Source | FDA Global Substance Registration System (GSRS) | |
URL | https://gsrs.ncats.nih.gov/ginas/app/beta/substances/U78804BIDR | |
Description | The FDA Global Substance Registration System (GSRS) enables the efficient and accurate exchange of information on what substances are in regulated products. Instead of relying on names, which vary across regulatory domains, countries, and regions, the GSRS knowledge base makes it possible for substances to be defined by standardized, scientific descriptions. | |
Explanation | Unless otherwise noted, the contents of the FDA website (www.fda.gov), both text and graphics, are not copyrighted. They are in the public domain and may be republished, reprinted and otherwise used freely by anyone without the need to obtain permission from FDA. Credit to the U.S. Food and Drug Administration as the source is appreciated but not required. | |
Retrosynthesis Analysis
AI-Powered Synthesis Planning: Our tool employs the Template_relevance Pistachio, Template_relevance Bkms_metabolic, Template_relevance Pistachio_ringbreaker, Template_relevance Reaxys, Template_relevance Reaxys_biocatalysis model, leveraging a vast database of chemical reactions to predict feasible synthetic routes.
One-Step Synthesis Focus: Specifically designed for one-step synthesis, it provides concise and direct routes for your target compounds, streamlining the synthesis process.
Accurate Predictions: Utilizing the extensive PISTACHIO, BKMS_METABOLIC, PISTACHIO_RINGBREAKER, REAXYS, REAXYS_BIOCATALYSIS database, our tool offers high-accuracy predictions, reflecting the latest in chemical research and data.
Strategy Settings
Precursor scoring | Relevance Heuristic |
---|---|
Min. plausibility | 0.01 |
Model | Template_relevance |
Template Set | Pistachio/Bkms_metabolic/Pistachio_ringbreaker/Reaxys/Reaxys_biocatalysis |
Top-N result to add to graph | 6 |
Feasible Synthetic Routes
Disclaimer and Information on In-Vitro Research Products
Please be aware that all articles and product information presented on BenchChem are intended solely for informational purposes. The products available for purchase on BenchChem are specifically designed for in-vitro studies, which are conducted outside of living organisms. In-vitro studies, derived from the Latin term "in glass," involve experiments performed in controlled laboratory settings using cells or tissues. It is important to note that these products are not categorized as medicines or drugs, and they have not received approval from the FDA for the prevention, treatment, or cure of any medical condition, ailment, or disease. We must emphasize that any form of bodily introduction of these products into humans or animals is strictly prohibited by law. It is essential to adhere to these guidelines to ensure compliance with legal and ethical standards in research and experimentation.