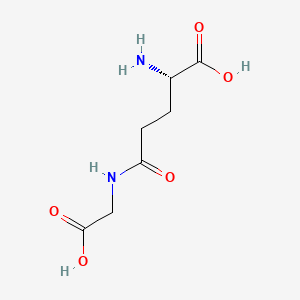
gamma-D-Glutamylglycine
Overview
Description
Gamma-D-Glutamylglycine: is a dipeptide composed of gamma-glutamyl and glycine residues. It is known for its role as an excitatory amino acid receptor antagonist, with a structure similar to gamma-aminobutyric acid (GABA)
Mechanism of Action
Target of Action
Gamma-D-Glutamylglycine (Gamma-DGG) is an excitatory amino acid receptor antagonist with a structure similar to gamma-aminobutyric acid (GABA) . It primarily targets G-cells in the stomach, which are responsible for secreting gastrin .
Mode of Action
Gamma-DGG interacts with its targets by inhibiting the action of ionotropic glutamate receptors , specifically acting as a low-affinity competitive antagonist at AMPA receptors . This means it competes with the neurotransmitter glutamate for binding sites on the AMPA receptors, thereby reducing the excitatory effect of glutamate.
Biochemical Pathways
Gamma-DGG is involved in the gamma-glutamyl cycle , a critical biochemical pathway that regulates the cellular levels of the antioxidant molecule glutathione . This cycle involves the enzyme gamma-glutamyl transpeptidase (γ-GT), which cleaves the γ-glutamyl amide bond of glutathione to give cysteinylglycine . The released γ-glutamyl group can be transferred to water (hydrolysis) or to amino acids or short peptides (transpeptidation) .
Pharmacokinetics
It’s known that dipeptides like gamma-dgg are absorbed more rapidly than amino acids because their uptake involves a separate mechanism .
Result of Action
The primary result of Gamma-DGG’s action is the reduction of excitatory neurotransmission, due to its antagonistic effect on AMPA receptors . This can have various downstream effects depending on the specific context, potentially influencing processes such as neuronal signaling, memory formation, and pain perception.
Biochemical Analysis
Biochemical Properties
Gamma-D-Glutamylglycine plays a crucial role in biochemical reactions, particularly in the modulation of excitatory amino acid receptors. It interacts with enzymes such as gamma-glutamyl transpeptidase, which cleaves the gamma-glutamyl bond to release the gamma-glutamyl group. This interaction is essential for the regulation of cellular levels of glutathione, an important antioxidant molecule. This compound also interacts with proteins involved in the gamma-glutamyl cycle, contributing to the maintenance of cellular redox homeostasis .
Cellular Effects
This compound influences various cellular processes, including cell signaling pathways, gene expression, and cellular metabolism. It has been shown to affect the phosphorylation of AMP-activated protein kinase (AMPK), promoting the nuclear translocation of glyceraldehyde-3-phosphate dehydrogenase (GAPDH) and inhibiting the nuclear translocation of deleted in breast cancer 1 (DBC1). These actions lead to the activation of sirtuin 1 (SIRT1) and the deacetylation of p53 in the nucleus, which are critical for cellular function and longevity .
Molecular Mechanism
At the molecular level, this compound exerts its effects through binding interactions with biomolecules and enzyme modulation. It acts as an antagonist to excitatory amino acid receptors, inhibiting their activity and thereby modulating neurotransmission. This inhibition is crucial for preventing excitotoxicity, a condition that can lead to neuronal damage. This compound also influences gene expression by modulating the activity of transcription factors and enzymes involved in cellular stress responses .
Temporal Effects in Laboratory Settings
In laboratory settings, the effects of this compound have been observed to change over time. The compound is relatively stable, but its activity can be influenced by factors such as temperature and pH. Long-term studies have shown that this compound can have sustained effects on cellular function, including the maintenance of redox balance and the prevention of oxidative stress .
Dosage Effects in Animal Models
The effects of this compound vary with different dosages in animal models. At low doses, it has been shown to have protective effects against oxidative stress and inflammation. At high doses, this compound can exhibit toxic effects, including disruptions in cellular metabolism and increased oxidative damage. These findings highlight the importance of dosage optimization in therapeutic applications .
Metabolic Pathways
This compound is involved in several metabolic pathways, including the gamma-glutamyl cycle. It interacts with enzymes such as gamma-glutamyl transpeptidase and dipeptidyl peptidase, which are crucial for its metabolism and function. These interactions influence metabolic flux and the levels of various metabolites, contributing to the overall metabolic balance within cells .
Transport and Distribution
Within cells and tissues, this compound is transported and distributed through specific transporters and binding proteins. These mechanisms ensure its proper localization and accumulation in target areas, where it can exert its biochemical effects. The transport and distribution of this compound are essential for its role in cellular homeostasis and function .
Subcellular Localization
This compound is localized in various subcellular compartments, including the cytoplasm and mitochondria. Its activity and function are influenced by its localization, with specific targeting signals and post-translational modifications directing it to these compartments. This subcellular localization is critical for the compound’s role in maintaining cellular redox balance and preventing oxidative stress .
Preparation Methods
Synthetic Routes and Reaction Conditions: Gamma-D-Glutamylglycine can be synthesized using bacterial gamma-glutamyltranspeptidase. This method involves the transfer of the gamma-glutamyl moiety from donor substrates, such as glutathione or glutamine, to an acceptor substrate, which can be water (hydrolysis) or other amino acids/small peptides (transpeptidation) . The reaction does not require modifications of reactive groups of the substrate or energy sources like ATP, making it an efficient and straightforward method .
Industrial Production Methods: The industrial production of this compound typically involves the use of bacterial gamma-glutamyltranspeptidase, which is readily available from strains over-producing this enzyme . This method is advantageous due to its simplicity and the wide range of gamma-glutamyl compounds that can be synthesized.
Chemical Reactions Analysis
Types of Reactions: Gamma-D-Glutamylglycine undergoes various chemical reactions, including hydrolysis and transpeptidation. It acts as an antagonist to excitatory amino acids in the hippocampus, suppressing depolarizations induced by L-aspartate, D-homocysteate, or L-glutamate .
Common Reagents and Conditions: The reactions involving this compound typically use gamma-glutamyltranspeptidase as a catalyst. The conditions for these reactions are relatively mild, not requiring high temperatures or pressures .
Major Products: The major products formed from the reactions of this compound include various gamma-glutamyl peptides and amino acids, depending on the acceptor substrates used in the reactions .
Scientific Research Applications
Gamma-D-Glutamylglycine has several scientific research applications, particularly in the fields of chemistry, biology, medicine, and industry. It is used as an excitatory amino acid receptor antagonist, making it valuable in neuroscience research . Additionally, its role in the gamma-glutamyl cycle and its ability to regulate cellular levels of glutathione make it significant in studies related to oxidative stress and cellular redox homeostasis .
Comparison with Similar Compounds
- Gamma-aminobutyric acid (GABA)
- Gamma-D-Glutamyl-L-Tryptophan
- Gamma-Glutamylcysteine
Comparison: Gamma-D-Glutamylglycine is unique in its structure and function as an excitatory amino acid receptor antagonist. Unlike gamma-aminobutyric acid, which is an inhibitory neurotransmitter, this compound specifically antagonizes excitatory amino acids . Gamma-D-Glutamyl-L-Tryptophan, on the other hand, is an immunomodulatory dipeptide with broad-spectrum stimulatory activities . Gamma-Glutamylcysteine is a precursor in the biosynthesis of glutathione and plays a crucial role in cellular redox homeostasis .
Properties
IUPAC Name |
(2R)-2-amino-5-(carboxymethylamino)-5-oxopentanoic acid | |
---|---|---|
Source | PubChem | |
URL | https://pubchem.ncbi.nlm.nih.gov | |
Description | Data deposited in or computed by PubChem | |
InChI |
InChI=1S/C7H12N2O5/c8-4(7(13)14)1-2-5(10)9-3-6(11)12/h4H,1-3,8H2,(H,9,10)(H,11,12)(H,13,14)/t4-/m1/s1 | |
Source | PubChem | |
URL | https://pubchem.ncbi.nlm.nih.gov | |
Description | Data deposited in or computed by PubChem | |
InChI Key |
ACIJGUBIMXQCMF-SCSAIBSYSA-N | |
Source | PubChem | |
URL | https://pubchem.ncbi.nlm.nih.gov | |
Description | Data deposited in or computed by PubChem | |
Canonical SMILES |
C(CC(=O)NCC(=O)O)C(C(=O)O)N | |
Source | PubChem | |
URL | https://pubchem.ncbi.nlm.nih.gov | |
Description | Data deposited in or computed by PubChem | |
Isomeric SMILES |
C(CC(=O)NCC(=O)O)[C@H](C(=O)O)N | |
Source | PubChem | |
URL | https://pubchem.ncbi.nlm.nih.gov | |
Description | Data deposited in or computed by PubChem | |
Molecular Formula |
C7H12N2O5 | |
Source | PubChem | |
URL | https://pubchem.ncbi.nlm.nih.gov | |
Description | Data deposited in or computed by PubChem | |
Molecular Weight |
204.18 g/mol | |
Source | PubChem | |
URL | https://pubchem.ncbi.nlm.nih.gov | |
Description | Data deposited in or computed by PubChem | |
CAS No. |
6729-55-1 | |
Record name | γ-D-Glutamylglycine | |
Source | CAS Common Chemistry | |
URL | https://commonchemistry.cas.org/detail?cas_rn=6729-55-1 | |
Description | CAS Common Chemistry is an open community resource for accessing chemical information. Nearly 500,000 chemical substances from CAS REGISTRY cover areas of community interest, including common and frequently regulated chemicals, and those relevant to high school and undergraduate chemistry classes. This chemical information, curated by our expert scientists, is provided in alignment with our mission as a division of the American Chemical Society. | |
Explanation | The data from CAS Common Chemistry is provided under a CC-BY-NC 4.0 license, unless otherwise stated. | |
Retrosynthesis Analysis
AI-Powered Synthesis Planning: Our tool employs the Template_relevance Pistachio, Template_relevance Bkms_metabolic, Template_relevance Pistachio_ringbreaker, Template_relevance Reaxys, Template_relevance Reaxys_biocatalysis model, leveraging a vast database of chemical reactions to predict feasible synthetic routes.
One-Step Synthesis Focus: Specifically designed for one-step synthesis, it provides concise and direct routes for your target compounds, streamlining the synthesis process.
Accurate Predictions: Utilizing the extensive PISTACHIO, BKMS_METABOLIC, PISTACHIO_RINGBREAKER, REAXYS, REAXYS_BIOCATALYSIS database, our tool offers high-accuracy predictions, reflecting the latest in chemical research and data.
Strategy Settings
Precursor scoring | Relevance Heuristic |
---|---|
Min. plausibility | 0.01 |
Model | Template_relevance |
Template Set | Pistachio/Bkms_metabolic/Pistachio_ringbreaker/Reaxys/Reaxys_biocatalysis |
Top-N result to add to graph | 6 |
Feasible Synthetic Routes
Q1: How does gamma-D-glutamylglycine (gamma-DGG) exert its antagonistic effects on excitatory amino acid receptors?
A1: gamma-DGG acts as a competitive antagonist at kainate and quisqualate receptors. [, ] This means it binds to these receptors, preventing the binding of endogenous agonists like glutamate and kainate, thus inhibiting their excitatory effects on neurons. [, ]
Q2: What is the evidence suggesting gamma-DGG's selectivity for kainate and quisqualate receptors over NMDA receptors?
A2: Several studies have demonstrated that gamma-DGG preferentially antagonizes kainate and quisqualate-induced responses in neurons, while having minimal effects on NMDA receptor-mediated responses. [, , , , ] For instance, in rat hippocampal slices, gamma-DGG effectively blocked depolarizations induced by kainate and quisqualate but showed little to no effect on NMDA-induced depolarizations. [] Similarly, in studies on frog spinal motoneurons, gamma-DGG suppressed glutamate currents evoked at resting potentials, suggesting its action on non-NMDA receptors. []
Q3: Does gamma-DGG affect synaptic transmission? If so, how?
A3: Yes, gamma-DGG has been shown to depress synaptic transmission in various brain regions, including the hippocampus, dentate gyrus, and visual cortex. [, , , , ] This effect is attributed to its blockade of postsynaptic kainate and quisqualate receptors, which are involved in mediating excitatory synaptic responses. [, ] For example, gamma-DGG effectively inhibited perforant path-evoked potentials in the dentate gyrus, even at concentrations that did not completely block the evoked potentials. []
Q4: Are there instances where gamma-DGG's effects on synaptic transmission appear to be independent of its action on glutamate or aspartate receptors?
A4: Yes, some studies have reported that gamma-DGG can depress synaptic excitation in the absence of a direct effect on glutamate or aspartate-induced excitation. [] This suggests that gamma-DGG might interact with other, yet unidentified, neurotransmitter systems or receptor subtypes involved in synaptic transmission.
Q5: Can gamma-DGG cross the blood-brain barrier?
A5: While the provided research doesn't directly address this question, the fact that gamma-DGG effectively antagonizes excitatory transmission in in vivo studies suggests it can at least partially cross the blood-brain barrier to exert its effects within the central nervous system. [, , , ]
Q6: What structural features of gamma-DGG are crucial for its antagonistic activity?
A6: The presence of the gamma-glutamyl moiety appears essential for gamma-DGG's antagonistic activity at excitatory amino acid receptors. [] This is supported by the observation that other compounds possessing this moiety, such as kainic acid and gamma-D-glutamylaminomethyl sulfonate (GAMS), also exhibit antagonistic effects on these receptors. [, ] Further research is needed to delineate the specific structural features responsible for gamma-DGG's selectivity profile and potency compared to other antagonists.
Q7: How has gamma-DGG been used in research to study excitatory neurotransmission?
A7: gamma-DGG has proven to be a valuable pharmacological tool in dissecting the roles of kainate and quisqualate receptors in various physiological and pathological processes. [, , , , ] For example, researchers have used gamma-DGG to:
- Investigate the contribution of non-NMDA receptors to long-term potentiation (LTP): Studies using gamma-DGG have provided insights into the role of non-NMDA receptors in synaptic plasticity, a process crucial for learning and memory. []
- Explore the involvement of excitatory amino acid receptors in different brain regions: By applying gamma-DGG to specific brain areas, researchers have gained a better understanding of the distribution and function of kainate and quisqualate receptors in mediating diverse neuronal circuits and behaviors. [, , ]
- Study the potential neuroprotective effects of blocking excitatory transmission: Research using gamma-DGG has contributed to understanding the role of excessive excitatory amino acid release in neuronal damage following hypoxic or ischemic insults. []
Q8: What are some of the limitations of using gamma-DGG as a pharmacological tool?
A8: Despite its usefulness, gamma-DGG has some limitations:
Disclaimer and Information on In-Vitro Research Products
Please be aware that all articles and product information presented on BenchChem are intended solely for informational purposes. The products available for purchase on BenchChem are specifically designed for in-vitro studies, which are conducted outside of living organisms. In-vitro studies, derived from the Latin term "in glass," involve experiments performed in controlled laboratory settings using cells or tissues. It is important to note that these products are not categorized as medicines or drugs, and they have not received approval from the FDA for the prevention, treatment, or cure of any medical condition, ailment, or disease. We must emphasize that any form of bodily introduction of these products into humans or animals is strictly prohibited by law. It is essential to adhere to these guidelines to ensure compliance with legal and ethical standards in research and experimentation.