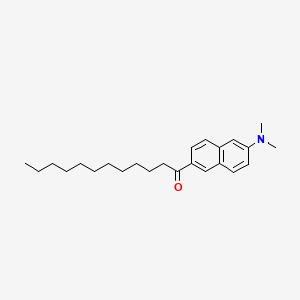
Laurdan
Overview
Description
Laurdan, also known as 6-lauroyl-2-(dimethylamino)naphthalene, is an organic compound widely used as a fluorescent dye in fluorescence microscopy. It was first synthesized in 1979 by the Argentinian scientist Gregorio Weber. This compound is particularly valuable for investigating the qualities of phospholipid bilayers in cell membranes, such as membrane fluidity and phase transitions .
Mechanism of Action
Target of Action
Laurdan, or 6-dodecanoyl-2-dimethylaminonaphthalene, is a synthetic, environmentally sensitive dye . Its primary target is the cellular membrane , specifically the lipid bilayer . The functional group of this compound is physically located at the carbonyl region of the bilayer . Due to its amphiphilic nature, this compound can insert into lipid bilayers at different depths and orientations .
Mode of Action
This compound’s interaction with its target results in changes in membrane fluidity . Membrane fluidity is a critical parameter of cellular membranes, which cells continuously strive to maintain within a viable range . An interference with the correct membrane fluidity state can strongly inhibit cell function . This compound’s fluorescence emission spectrum is sensitive to the presence of H2O close to its chromophore , allowing it to detect changes in membrane fluidity.
Biochemical Pathways
This compound’s action primarily affects the biochemical pathways related to membrane fluidity and permeability . By interacting with the lipid bilayer, this compound can influence the diffusion barrier function of the cytoplasmic membrane . This can lead to changes in the overall membrane fluidity or trigger the formation of abnormal lipid domains , potentially inhibiting cell growth .
Pharmacokinetics
It is known that this compound is essentially nonfluorescent in water but becomes fluorescent when incorporated into a lipid bilayer .
Result of Action
The primary result of this compound’s action is the alteration of membrane fluidity . This can lead to changes in the overall membrane fluidity or the formation of abnormal lipid domains . These changes can strongly inhibit cell function , affecting a multitude of membrane-associated cellular processes .
Action Environment
The action of this compound can be influenced by various environmental factors. For instance, cholesterol levels in lipid vesicles can alter membrane hydration and viscosity , affecting this compound’s action. Moreover, this compound is designed to stain exclusively the outer leaflet of lipid bilayers , making it more sensitive to changes in the external environment.
Biochemical Analysis
Biochemical Properties
Laurdan’s fluorescence properties are sensitive to the polarity of its environment . This sensitivity arises from the large increase in the molecule’s dipole moment in the fluorescence state over that of the ground state . In the context of biochemical reactions, this compound interacts with lipids in the membrane, with its optical properties changing depending on the phase and temperature of the membrane .
Cellular Effects
This compound’s fluorescence properties allow it to report on the hydration status and phase of lipid bilayers, which are key determinants of cell function . Changes in this compound’s fluorescence can indicate alterations in cell signaling pathways, gene expression, and cellular metabolism, as these processes can influence the organization and dynamics of the lipid bilayer .
Molecular Mechanism
This compound exerts its effects at the molecular level through its interactions with lipids in the membrane . Its fluorescence properties change in response to alterations in the polarity of its environment, which can occur due to changes in lipid packing and hydration . This allows this compound to act as a reporter of membrane dynamics and organization .
Temporal Effects in Laboratory Settings
The effects of this compound can change over time in laboratory settings, as its fluorescence properties are sensitive to the temperature and phase of the membrane . For example, molecular dynamics simulations have shown that the fluorescence lifetime and anisotropy of this compound can be used to identify different membrane phases .
Metabolic Pathways
This compound itself is not involved in metabolic pathways, but its fluorescence properties can provide insights into the effects of metabolic processes on the organization and dynamics of the lipid bilayer .
Transport and Distribution
This compound is incorporated into membranes, where it interacts with lipids . Its distribution within cells and tissues would therefore be expected to reflect the distribution of these lipids.
Subcellular Localization
The subcellular localization of this compound would be determined by the distribution of the lipids with which it interacts . Its fluorescence properties could potentially be used to study the dynamics of specific compartments or organelles, provided these structures contain the appropriate lipids .
Preparation Methods
Laurdan is synthesized through a multi-step process involving the reaction of lauric acid with 2-(dimethylamino)naphthalene. The synthetic route typically includes the following steps:
Esterification: Lauric acid is esterified with methanol to form methyl laurate.
Friedel-Crafts Acylation: Methyl laurate undergoes Friedel-Crafts acylation with 2-(dimethylamino)naphthalene in the presence of a Lewis acid catalyst, such as aluminum chloride, to form this compound.
Purification: The crude product is purified using column chromatography to obtain pure this compound.
Industrial production methods for this compound are similar to laboratory synthesis but are scaled up to meet commercial demands. The reaction conditions are optimized for higher yields and purity, often involving automated processes and advanced purification techniques.
Chemical Reactions Analysis
Laurdan undergoes various chemical reactions, including:
Oxidation: this compound can be oxidized using strong oxidizing agents like potassium permanganate, leading to the formation of naphthoquinone derivatives.
Reduction: Reduction of this compound can be achieved using reducing agents such as sodium borohydride, resulting in the formation of reduced naphthalene derivatives.
Substitution: this compound can undergo substitution reactions, particularly at the dimethylamino group, using reagents like alkyl halides to form quaternary ammonium salts.
Common reagents and conditions used in these reactions include organic solvents like dichloromethane, acetonitrile, and methanol, along with catalysts and temperature control to ensure optimal reaction rates and yields .
Scientific Research Applications
Laurdan has a wide range of scientific research applications, including:
Chemistry: this compound is used as a probe to study the polarity and dynamics of various chemical environments.
Biology: In biological research, this compound is employed to investigate membrane fluidity, lipid phase transitions, and the organization of lipid domains in cell membranes.
Medicine: this compound is used in medical research to study the effects of drugs on cell membranes and to investigate membrane-related diseases.
Comparison with Similar Compounds
Laurdan is often compared with other environmentally sensitive dyes, such as PRODAN (6-propionyl-2-(dimethylamino)naphthalene) and ACDAN (6-acetyl-2-(dimethylamino)naphthalene). While all these compounds share a similar naphthalene structure, this compound is unique in its ability to study membrane fluidity and phase transitions due to its longer lauroyl chain, which enhances its integration into lipid bilayers .
Similar compounds include:
PRODAN: Used for studying solvent polarity and protein-lipid interactions.
ACDAN: Employed in fluorescence microscopy for investigating membrane dynamics.
This compound’s distinct properties make it a valuable tool in various fields of scientific research, providing insights into the dynamic behavior of biological membranes and other complex systems.
Properties
IUPAC Name |
1-[6-(dimethylamino)naphthalen-2-yl]dodecan-1-one | |
---|---|---|
Source | PubChem | |
URL | https://pubchem.ncbi.nlm.nih.gov | |
Description | Data deposited in or computed by PubChem | |
InChI |
InChI=1S/C24H35NO/c1-4-5-6-7-8-9-10-11-12-13-24(26)22-15-14-21-19-23(25(2)3)17-16-20(21)18-22/h14-19H,4-13H2,1-3H3 | |
Source | PubChem | |
URL | https://pubchem.ncbi.nlm.nih.gov | |
Description | Data deposited in or computed by PubChem | |
InChI Key |
JHDGGIDITFLRJY-UHFFFAOYSA-N | |
Source | PubChem | |
URL | https://pubchem.ncbi.nlm.nih.gov | |
Description | Data deposited in or computed by PubChem | |
Canonical SMILES |
CCCCCCCCCCCC(=O)C1=CC2=C(C=C1)C=C(C=C2)N(C)C | |
Source | PubChem | |
URL | https://pubchem.ncbi.nlm.nih.gov | |
Description | Data deposited in or computed by PubChem | |
Molecular Formula |
C24H35NO | |
Source | PubChem | |
URL | https://pubchem.ncbi.nlm.nih.gov | |
Description | Data deposited in or computed by PubChem | |
DSSTOX Substance ID |
DTXSID80225517 | |
Record name | Laurdan | |
Source | EPA DSSTox | |
URL | https://comptox.epa.gov/dashboard/DTXSID80225517 | |
Description | DSSTox provides a high quality public chemistry resource for supporting improved predictive toxicology. | |
Molecular Weight |
353.5 g/mol | |
Source | PubChem | |
URL | https://pubchem.ncbi.nlm.nih.gov | |
Description | Data deposited in or computed by PubChem | |
CAS No. |
74515-25-6 | |
Record name | 1-[6-(Dimethylamino)-2-naphthalenyl]-1-dodecanone | |
Source | CAS Common Chemistry | |
URL | https://commonchemistry.cas.org/detail?cas_rn=74515-25-6 | |
Description | CAS Common Chemistry is an open community resource for accessing chemical information. Nearly 500,000 chemical substances from CAS REGISTRY cover areas of community interest, including common and frequently regulated chemicals, and those relevant to high school and undergraduate chemistry classes. This chemical information, curated by our expert scientists, is provided in alignment with our mission as a division of the American Chemical Society. | |
Explanation | The data from CAS Common Chemistry is provided under a CC-BY-NC 4.0 license, unless otherwise stated. | |
Record name | Laurdan | |
Source | ChemIDplus | |
URL | https://pubchem.ncbi.nlm.nih.gov/substance/?source=chemidplus&sourceid=0074515256 | |
Description | ChemIDplus is a free, web search system that provides access to the structure and nomenclature authority files used for the identification of chemical substances cited in National Library of Medicine (NLM) databases, including the TOXNET system. | |
Record name | Laurdan | |
Source | EPA DSSTox | |
URL | https://comptox.epa.gov/dashboard/DTXSID80225517 | |
Description | DSSTox provides a high quality public chemistry resource for supporting improved predictive toxicology. | |
Record name | LAURDAN | |
Source | FDA Global Substance Registration System (GSRS) | |
URL | https://gsrs.ncats.nih.gov/ginas/app/beta/substances/Y97FBL93VW | |
Description | The FDA Global Substance Registration System (GSRS) enables the efficient and accurate exchange of information on what substances are in regulated products. Instead of relying on names, which vary across regulatory domains, countries, and regions, the GSRS knowledge base makes it possible for substances to be defined by standardized, scientific descriptions. | |
Explanation | Unless otherwise noted, the contents of the FDA website (www.fda.gov), both text and graphics, are not copyrighted. They are in the public domain and may be republished, reprinted and otherwise used freely by anyone without the need to obtain permission from FDA. Credit to the U.S. Food and Drug Administration as the source is appreciated but not required. | |
Synthesis routes and methods
Procedure details
Retrosynthesis Analysis
AI-Powered Synthesis Planning: Our tool employs the Template_relevance Pistachio, Template_relevance Bkms_metabolic, Template_relevance Pistachio_ringbreaker, Template_relevance Reaxys, Template_relevance Reaxys_biocatalysis model, leveraging a vast database of chemical reactions to predict feasible synthetic routes.
One-Step Synthesis Focus: Specifically designed for one-step synthesis, it provides concise and direct routes for your target compounds, streamlining the synthesis process.
Accurate Predictions: Utilizing the extensive PISTACHIO, BKMS_METABOLIC, PISTACHIO_RINGBREAKER, REAXYS, REAXYS_BIOCATALYSIS database, our tool offers high-accuracy predictions, reflecting the latest in chemical research and data.
Strategy Settings
Precursor scoring | Relevance Heuristic |
---|---|
Min. plausibility | 0.01 |
Model | Template_relevance |
Template Set | Pistachio/Bkms_metabolic/Pistachio_ringbreaker/Reaxys/Reaxys_biocatalysis |
Top-N result to add to graph | 6 |
Feasible Synthetic Routes
Disclaimer and Information on In-Vitro Research Products
Please be aware that all articles and product information presented on BenchChem are intended solely for informational purposes. The products available for purchase on BenchChem are specifically designed for in-vitro studies, which are conducted outside of living organisms. In-vitro studies, derived from the Latin term "in glass," involve experiments performed in controlled laboratory settings using cells or tissues. It is important to note that these products are not categorized as medicines or drugs, and they have not received approval from the FDA for the prevention, treatment, or cure of any medical condition, ailment, or disease. We must emphasize that any form of bodily introduction of these products into humans or animals is strictly prohibited by law. It is essential to adhere to these guidelines to ensure compliance with legal and ethical standards in research and experimentation.