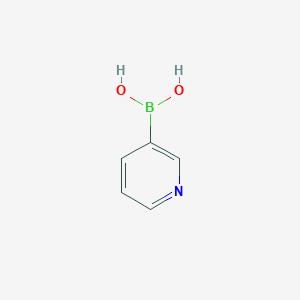
Pyridine-3-boronic acid
Overview
Description
Pyridine-3-boronic acid (C₅H₆BNO₂; CAS 1692-25-7) is a heterocyclic boronic acid with a boron atom attached to the 3-position of a pyridine ring. This compound is widely used in organic synthesis, particularly in Suzuki-Miyaura cross-coupling reactions, due to its ability to form stable covalent bonds with aryl halides under catalytic conditions . Its applications extend to medicinal chemistry, where it serves as a key scaffold in efflux pump inhibitors and sialic acid-binding agents .
Preparation Methods
Synthetic Routes and Reaction Conditions: The primary method for synthesizing pyridine-3-boronic acid involves the electrophilic trapping of an organometallic reagent with a boric ester, such as triisopropyl borate (B(OiPr)3) or trimethyl borate (B(OMe)3). This reaction is typically performed at low temperatures to prevent over-alkylation, which could lead to the formation of borinic esters instead of boronic acids .
Industrial Production Methods: Industrial production of this compound often employs similar synthetic routes but on a larger scale. The process involves careful control of reaction conditions to ensure high yield and purity. The use of continuous flow setups for handling organolithium chemistry on a multigram scale has also been reported, enabling efficient production of boronic acids .
Chemical Reactions Analysis
Suzuki-Miyaura Cross-Coupling Reactions
Pyridine-3-boronic acid is widely used in palladium-catalyzed Suzuki-Miyaura couplings to form biaryl or heteroaryl structures. Representative examples include:
Table 1: Suzuki-Miyaura Coupling of this compound
Key observations:
-
Catalyst choice significantly impacts efficiency. Pd(dppf)Cl₂ achieves higher yields (74.6%) compared to PEPPSI-IPr (40.2%) under similar conditions .
-
Solvent systems like 1,4-dioxane/water or THF/water are optimal for balancing reactivity and solubility .
Formation of Dihydropyridine Boronic Esters
Activation of this compound pinacol ester with acylating agents enables reactions with organometallic reagents:
Mechanism :
-
N-Acylation of the pyridine ring.
-
Nucleophilic attack by organometallic reagents (e.g., tert-BuLi) at the C2 position.
-
Formation of dihydropyridine boronic esters (yields: 58–85% ) .
Applications :
pH-Dependent Reactivity and Stability
The boronic acid group and pyridine ring exhibit pH-sensitive behavior:
Acidity Studies :
-
The boron center in PBA has a pKₐ of 4.4 , while the pyridinium moiety’s acidity increases with substituents (e.g., methyl groups lower pKₐ) .
-
Under acidic conditions, protonation of the pyridine nitrogen enhances electrophilicity, accelerating reactions like complexation with 4-isopropyltropolone .
Peroxide Stability :
Scientific Research Applications
Medicinal Chemistry
Anticancer Applications
Pyridine-3-boronic acid is recognized for its potential in developing anticancer therapies. It has been shown to selectively bind to sialic acids (SAs), which are often overexpressed in cancer cells. This property enhances the targeting of tumor cells, making PBA a candidate for drug delivery systems aimed at improving therapeutic outcomes in cancer treatment. For instance, derivatives such as 5-boronopicolinic acid exhibit high binding constants with SAs under acidic conditions typical of tumor microenvironments, facilitating targeted delivery of chemotherapeutics .
Antibacterial and Antiviral Activity
Research indicates that boronic acids, including PBA, possess antibacterial and antiviral properties. They can inhibit multidrug efflux pumps in bacteria, enhancing the efficacy of existing antibiotics. Studies have shown that PBA derivatives can be effective against resistant strains of Staphylococcus aureus by disrupting their efflux mechanisms .
Organic Synthesis
Suzuki-Miyaura Cross-Coupling Reactions
this compound serves as a key reagent in Suzuki-Miyaura cross-coupling reactions, which are fundamental in organic synthesis for forming carbon-carbon bonds. Its ability to participate in these reactions allows for the construction of complex organic molecules, including pharmaceuticals and agrochemicals. The regioselectivity and efficiency of these reactions can be significantly enhanced using PBA as a coupling partner .
Microwave-Assisted Synthesis
Recent advancements have demonstrated that PBA can be utilized effectively in microwave-assisted synthetic protocols. This method reduces reaction times and minimizes side product formation, making it an attractive option for large-scale synthesis .
Sensor Development
This compound has been employed in the development of sensors due to its ability to form reversible covalent bonds with diols and sugars. These properties make it suitable for detecting biomolecules such as glucose and other saccharides, which is particularly useful in medical diagnostics .
Material Science
Catalysts and Polymerization
In material science, PBA is utilized as a catalyst in various polymerization reactions. Its boron atom facilitates the formation of boronate esters, which are crucial intermediates in synthesizing polymers with specific functionalities . Additionally, PBA derivatives have been explored for their roles in creating functionalized materials with applications ranging from drug delivery systems to smart materials.
Table 1: Biological Activities of this compound Derivatives
Compound Name | Activity Type | Target | Reference |
---|---|---|---|
5-Boronopicolinic Acid | Anticancer | Sialic Acid Binding | |
N-Diethylaminoboronate | Antibacterial | Staphylococcus aureus | |
Vaborbactam | Antiviral | β-lactamase Inhibition |
Table 2: Synthetic Applications of this compound
Application | Description | Reference |
---|---|---|
Suzuki-Miyaura Coupling | Formation of carbon-carbon bonds | |
Microwave-Assisted Synthesis | Accelerated reaction times | |
Sensor Development | Detection of glucose and biomolecules |
Case Studies
Case Study 1: Targeted Drug Delivery
A study investigated the use of 5-boronopicolinic acid for delivering anticancer drugs to pancreatic cancer cells. The compound demonstrated significant binding affinity to sialylated glycoconjugates on the cell surface, leading to enhanced drug uptake and reduced side effects compared to conventional delivery methods .
Case Study 2: Antibiotic Resistance
Research focused on the efficacy of this compound derivatives against methicillin-resistant Staphylococcus aureus (MRSA). The study revealed that these derivatives could potentiate the effects of existing antibiotics by inhibiting efflux pumps, providing a promising strategy for overcoming antibiotic resistance .
Mechanism of Action
The mechanism of action of pyridine-3-boronic acid in cross-coupling reactions involves the formation of a stable transition complex with the palladium catalyst. This complex facilitates the transfer of the boronic acid group to the electrophilic partner, forming a new carbon-carbon bond . In biological applications, this compound can form stable complexes with sugars and amino acids, inhibiting specific molecular targets such as efflux pumps in bacteria .
Comparison with Similar Compounds
Structural and Electronic Differences
Pyridine-3-boronic acid is distinguished from its isomers (e.g., pyridine-2- or -4-boronic acid) by the position of the boronic acid group on the pyridine ring. This positional variation significantly impacts electronic properties:
- This compound : The boron at the 3-position interacts with the nitrogen atom in the ring, creating a meta-directing effect that stabilizes intermediates in cross-coupling reactions .
- Pyridine-4-boronic acid : The boron at the 4-position (para to nitrogen) exhibits weaker electronic conjugation with the nitrogen, leading to reduced reactivity in some catalytic systems .
Table 1: Structural Comparison of Pyridine Boronic Acid Isomers
Table 2: Efflux Pump Inhibition by this compound Derivatives
Sialic Acid (SA) Binding in Acidic Environments
This compound derivatives exhibit pH-dependent binding to sialic acids, relevant to tumor microenvironments (pH ~6.5):
- (6-Propylcarbamoyl)this compound: Maintains a binding constant (K) of ~1,200 M⁻¹ at pH 6.5, comparable to 5-boronopicolinic acid (K = 1,300 M⁻¹) .
- 3-Pyridylboronic acid (unsubstituted) : Shows weaker binding (K = 800 M⁻¹ at pH 6.5), highlighting the importance of functionalization .
Table 3: Sialic Acid Binding Constants at pH 6.5
Reactivity in Cross-Coupling Reactions
This compound is highly reactive in CuCl-catalyzed oxidative self-coupling, outperforming sterically hindered derivatives:
Biological Activity
Pyridine-3-boronic acid (CHBNO) is a compound of significant interest in medicinal chemistry due to its diverse biological activities, particularly in the fields of antibacterial, anticancer, and antiviral research. This article explores the biological activity of this compound, synthesizing findings from various studies, and presenting them in a structured format.
Overview of this compound
This compound is characterized by its boronic acid functional group attached to a pyridine ring. This structural configuration allows for unique interactions with biological targets, making it a valuable scaffold in drug development.
Antibacterial Activity
This compound and its derivatives have been investigated for their antibacterial properties. Notably, studies have shown that certain derivatives can potentiate the activity of existing antibiotics.
Key Findings:
- Enhancement of Antibiotic Efficacy : Compounds such as 6-(3-phenylpropoxy)this compound were found to enhance the efficacy of ciprofloxacin against Staphylococcus aureus by fourfold, indicating their potential as efflux pump inhibitors .
- Mechanism of Action : The proposed mechanism involves the inhibition of the NorA efflux pump, which is responsible for antibiotic resistance in certain bacterial strains .
Table: Antibacterial Activity of this compound Derivatives
Compound Name | Target Bacteria | Activity Enhancement | Mechanism of Action |
---|---|---|---|
6-(3-Phenylpropoxy)this compound | Staphylococcus aureus | 4-fold increase | NorA efflux pump inhibitor |
6-(4-phenylbutoxy)this compound | Staphylococcus aureus | Significant | NorA efflux pump inhibitor |
Anticancer Activity
Research has also highlighted the anticancer potential of this compound derivatives. These compounds have been shown to exhibit selective cytotoxicity against various cancer cell lines.
Key Findings:
- Proteasome Inhibition : Some boronic acids act as proteasome inhibitors, which can halt cancer cell proliferation by inducing cell cycle arrest at the G2/M phase .
- Combination Therapies : Studies indicate that combining this compound derivatives with established chemotherapeutics can enhance therapeutic efficacy while reducing resistance mechanisms .
Antiviral Activity
This compound has also been explored for its antiviral properties. Its ability to interact with viral proteins presents opportunities for developing antiviral agents.
Key Findings:
- Sialic Acid Binding : Certain derivatives exhibit selective binding to sialic acids under weakly acidic conditions, which is relevant for targeting viral infections that exploit sialic acid interactions .
- Potential Applications : These interactions suggest that this compound could be utilized in designing antiviral drugs, particularly for viruses that rely on sialic acid for entry into host cells.
Case Studies
- Antibacterial Study : A study focusing on the synthesis and evaluation of 3-(pyridine-3-yl)-2-oxazolidinone derivatives demonstrated significant antibacterial activity against multiple strains, confirming the role of pyridine derivatives in combating resistant bacteria .
- Anticancer Research : In a clinical trial setting, combinations involving bortezomib and pyridine-based compounds showed promising results in managing aggressive lymphomas, highlighting their potential in oncology .
Q & A
Basic Research Questions
Q. How can Pyridine-3-boronic acid be structurally identified, and what are its key physicochemical properties?
this compound (CAS 1692-25-7) is characterized by its molecular formula C₅H₆BNO₂ (MW 122.92 g/mol) and features a boronic acid group at the 3-position of the pyridine ring. Key identification methods include NMR (e.g., B NMR for boron environments) and FT-IR spectroscopy (B-O stretching at ~1340 cm⁻¹). Its white crystalline solid form and solubility in polar solvents (e.g., water, methanol) make it suitable for aqueous reactions. Safety data indicate no acute hazards, but handling requires precautions due to incomplete toxicological profiling .
Q. What are the primary applications of this compound in synthetic chemistry?
The compound is widely used in Suzuki-Miyaura cross-coupling reactions to introduce pyridyl groups into aromatic systems. For example, it reacts with aryl halides (e.g., 2-fluoro-5-bromopyridine) under palladium catalysis (Pd(OAc)₂, P(-tolyl)₃) in DME/water at 80°C to form biaryl products. This method is critical for synthesizing nicotinic acetylcholine receptor ligands and other heterocyclic pharmaceuticals .
Advanced Research Questions
Q. How does pH influence the binding affinity of this compound to biomolecules like sialic acid?
this compound binds sialic acid via reversible boronate ester formation, with binding constants () strongly pH-dependent. At pH 6.5 (mimicking tumor microenvironments), values remain high (~10³ M⁻¹), while neutral pH reduces affinity due to decreased boronic acid ionization. This pH sensitivity is exploited in tumor-targeting drug conjugates and sensors. Comparative studies show substituents (e.g., carboxymethyl groups at C-6) enhance binding stability under acidic conditions .
Q. What structural modifications optimize this compound for efflux pump modulation in antibacterial research?
Substituent positioning is critical: C-3 boronic acid derivatives exhibit potent NorA efflux pump inhibition in Staphylococcus aureus, whereas C-4 analogues are inactive. Introducing electron-withdrawing groups (e.g., benzyloxy at C-6) enhances activity by 4-fold. Cyclization at C-5/C-6 or methyl substitution at C-5 maintains potency, while sodium boronate salts reduce efficacy. These insights guide the design of non-cytotoxic adjuvants for antibiotics like ciprofloxacin .
Q. How can researchers address contradictions in reported binding constants for this compound across studies?
Discrepancies arise from variations in experimental conditions (pH, temperature, solvent polarity) and analytical methods (e.g., H NMR vs. fluorescence titration). Standardizing protocols (e.g., using pH 7.4 PBS buffer for physiological relevance) and validating with orthogonal techniques (ITC, SPR) improve reproducibility. Additionally, reporting anhydride content (common in commercial samples) is essential, as it affects reactivity .
Q. What methodologies improve the solubility and stability of this compound in aqueous systems?
N-Alkylation (e.g., triflate salts) or conjugation with hydrophilic groups (e.g., propylcarbamoyl) enhances water solubility while retaining binding activity. For micellar oxidative systems, alkylation at the pyridinium nitrogen improves compatibility with aqueous matrices. Alternatively, pinacol ester protection (boronate ester) stabilizes the compound during storage, with deprotection achievable via mild acid hydrolysis .
Q. How can boronic acid-functionalized hydrogels incorporating this compound be optimized for glucose sensing?
Incorporate this compound into poly(vinyl alcohol) hydrogels via boronate ester crosslinking. The hydrogel’s glucose sensitivity arises from competitive binding between glucose and the polymer diols. Optimizing the boronic acid/diol ratio and using zwitterionic co-monomers (e.g., sulfobetaine) enhance response kinetics. Validation via electrochemical impedance spectroscopy (EIS) confirms reversible swelling at physiological glucose concentrations (5–20 mM) .
Q. Methodological Considerations
Q. What experimental design principles are critical for studying this compound in cell-based assays?
- Hypoxia mimicry : Use CO₂-independent media buffered at pH 6.5 to replicate tumor microenvironments.
- Control for boronic acid reactivity : Include competitive diols (e.g., mannitol) to confirm target-specific binding.
- Cytotoxicity screening : Assess viability via MTT assays, as boronic acids may interfere with mitochondrial redox pathways .
Q. How should researchers validate the purity of this compound for reproducibility in catalysis?
Employ HPLC-MS to quantify anhydride impurities (common in boronic acids). Recrystallization from ethanol/water (1:3 v/v) removes residual anhydrides. Purity ≥95% is recommended for catalytic applications, verified via H NMR integration of the boronic acid proton (~8.5 ppm) .
Properties
IUPAC Name |
pyridin-3-ylboronic acid | |
---|---|---|
Source | PubChem | |
URL | https://pubchem.ncbi.nlm.nih.gov | |
Description | Data deposited in or computed by PubChem | |
InChI |
InChI=1S/C5H6BNO2/c8-6(9)5-2-1-3-7-4-5/h1-4,8-9H | |
Source | PubChem | |
URL | https://pubchem.ncbi.nlm.nih.gov | |
Description | Data deposited in or computed by PubChem | |
InChI Key |
ABMYEXAYWZJVOV-UHFFFAOYSA-N | |
Source | PubChem | |
URL | https://pubchem.ncbi.nlm.nih.gov | |
Description | Data deposited in or computed by PubChem | |
Canonical SMILES |
B(C1=CN=CC=C1)(O)O | |
Source | PubChem | |
URL | https://pubchem.ncbi.nlm.nih.gov | |
Description | Data deposited in or computed by PubChem | |
Molecular Formula |
C5H6BNO2 | |
Record name | 3-pyridinylboronic acid | |
Source | Wikipedia | |
URL | https://en.wikipedia.org/wiki/Dictionary_of_chemical_formulas | |
Description | Chemical information link to Wikipedia. | |
Source | PubChem | |
URL | https://pubchem.ncbi.nlm.nih.gov | |
Description | Data deposited in or computed by PubChem | |
DSSTOX Substance ID |
DTXSID30370268 | |
Record name | Pyridine-3-boronic acid | |
Source | EPA DSSTox | |
URL | https://comptox.epa.gov/dashboard/DTXSID30370268 | |
Description | DSSTox provides a high quality public chemistry resource for supporting improved predictive toxicology. | |
Molecular Weight |
122.92 g/mol | |
Source | PubChem | |
URL | https://pubchem.ncbi.nlm.nih.gov | |
Description | Data deposited in or computed by PubChem | |
CAS No. |
1692-25-7 | |
Record name | Pyridin-3-ylboronic acid | |
Source | CAS Common Chemistry | |
URL | https://commonchemistry.cas.org/detail?cas_rn=1692-25-7 | |
Description | CAS Common Chemistry is an open community resource for accessing chemical information. Nearly 500,000 chemical substances from CAS REGISTRY cover areas of community interest, including common and frequently regulated chemicals, and those relevant to high school and undergraduate chemistry classes. This chemical information, curated by our expert scientists, is provided in alignment with our mission as a division of the American Chemical Society. | |
Explanation | The data from CAS Common Chemistry is provided under a CC-BY-NC 4.0 license, unless otherwise stated. | |
Record name | Pyridine-3-boronic acid | |
Source | EPA DSSTox | |
URL | https://comptox.epa.gov/dashboard/DTXSID30370268 | |
Description | DSSTox provides a high quality public chemistry resource for supporting improved predictive toxicology. | |
Synthesis routes and methods I
Procedure details
Synthesis routes and methods II
Procedure details
Retrosynthesis Analysis
AI-Powered Synthesis Planning: Our tool employs the Template_relevance Pistachio, Template_relevance Bkms_metabolic, Template_relevance Pistachio_ringbreaker, Template_relevance Reaxys, Template_relevance Reaxys_biocatalysis model, leveraging a vast database of chemical reactions to predict feasible synthetic routes.
One-Step Synthesis Focus: Specifically designed for one-step synthesis, it provides concise and direct routes for your target compounds, streamlining the synthesis process.
Accurate Predictions: Utilizing the extensive PISTACHIO, BKMS_METABOLIC, PISTACHIO_RINGBREAKER, REAXYS, REAXYS_BIOCATALYSIS database, our tool offers high-accuracy predictions, reflecting the latest in chemical research and data.
Strategy Settings
Precursor scoring | Relevance Heuristic |
---|---|
Min. plausibility | 0.01 |
Model | Template_relevance |
Template Set | Pistachio/Bkms_metabolic/Pistachio_ringbreaker/Reaxys/Reaxys_biocatalysis |
Top-N result to add to graph | 6 |
Feasible Synthetic Routes
Disclaimer and Information on In-Vitro Research Products
Please be aware that all articles and product information presented on BenchChem are intended solely for informational purposes. The products available for purchase on BenchChem are specifically designed for in-vitro studies, which are conducted outside of living organisms. In-vitro studies, derived from the Latin term "in glass," involve experiments performed in controlled laboratory settings using cells or tissues. It is important to note that these products are not categorized as medicines or drugs, and they have not received approval from the FDA for the prevention, treatment, or cure of any medical condition, ailment, or disease. We must emphasize that any form of bodily introduction of these products into humans or animals is strictly prohibited by law. It is essential to adhere to these guidelines to ensure compliance with legal and ethical standards in research and experimentation.