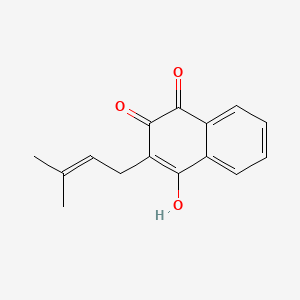
Lapachol
Overview
Description
Lapachol (2-hydroxy-3-(3-methyl-2-butenyl)-1,4-naphthoquinone) is a naturally occurring naphthoquinone isolated primarily from plants of the Bignoniaceae family, such as Tabebuia spp. and Handroanthus impetiginosus . It is characterized by a 1,4-naphthoquinone core with a prenyl side chain at the C-3 position, contributing to its redox-active properties and diverse biological activities . Historically recognized for its antitumor effects against Walker 256 carcinosarcoma , this compound has demonstrated anti-inflammatory, antimicrobial, antileishmanial, and antidiabetic properties in preclinical studies . Its mechanism of action includes modulation of oxidative stress pathways, inhibition of pro-inflammatory cytokines, and interaction with cellular targets like sirtuins (Sirt1/Sirt3) and ribosomal protein S6 kinase .
Preparation Methods
Traditional Extraction from Natural Sources
Lapachol is natively found in the heartwood of trees belonging to the Bignoniaceae family, notably Tabebuia species. Traditional extraction involves solvent-based isolation from powdered plant material. For instance, bark samples are macerated in ethanol or dichloromethane, followed by filtration and solvent evaporation to yield crude this compound. Subsequent purification steps, such as recrystallization from hot ethanol or chromatographic separation, enhance purity. Although this method preserves the compound’s natural configuration, it faces limitations in yield variability and scalability, driving the development of synthetic alternatives.
Synthetic Preparation Methods
One-Step Solvent-Free Synthesis
A novel solvent-free method for synthesizing this compound analogues was disclosed in the CN105503692A patent. While primarily focused on alpha-lapachol derivatives, this approach offers insights into optimizing naphthoquinone chemistry. The protocol involves:
- Reaction Setup : Lawsone (2-hydroxy-1,4-naphthoquinone) and nitrobenzaldehyde are combined with indium(III) chloride (InCl₃) as a catalyst.
- Solvent-Free Conditions : The mixture is stirred at elevated temperatures (80–100°C) without additional solvents, minimizing environmental impact.
- Product Isolation : Post-reaction, the crude product is washed with dichloromethane and purified via column chromatography.
This method highlights advancements in green chemistry, eliminating volatile organic solvents and reducing waste. However, its applicability to unmodified this compound requires further validation.
Multi-Step Synthesis with Indium(III) Chloride Catalyst
The EP1363896B1 patent delineates a robust multi-step synthesis of this compound, subsequently used to produce beta-lapachone. Key stages include:
Condensation Reaction :
- Naphthoquinone derivatives react with nitrobenzaldehyde in the presence of InCl₃.
- The mixture is stirred at room temperature, facilitating the formation of an intermediate Schiff base.
Extraction and Washing :
Drying and Evaporation :
- The organic layer is dried over sodium sulfate (Na₂SO₄), filtered, and concentrated via rotary evaporation.
- Residual toluene is co-evaporated with isopropanol to prevent solvent contamination.
Crystallization :
This method achieves high purity (>95%) and scalability, making it industrially viable.
Comparative Analysis of Synthetic Methods
The following table contrasts the two primary synthetic approaches:
Purification and Isolation Techniques
Post-synthetic purification is critical for obtaining pharmaceutical-grade this compound. The EP1363896B1 protocol employs a multi-solvent recrystallization strategy:
- Ethanol-Water System : Crude this compound is dissolved in a 3:1 ethanol-water mixture at 80°C.
- Graded Cooling : Slow cooling to 4°C promotes selective crystallization, excluding impurities.
- Filtration and Drying : Crystals are vacuum-filtered, washed with cold ethanol, and desiccated under reduced pressure.
This method yields a purity exceeding 98%, as confirmed by high-performance liquid chromatography (HPLC).
Environmental and Industrial Considerations
Synthetic routes utilizing InCl₃, though efficient, pose challenges due to indium’s cost and limited availability. Recent efforts explore substituting InCl₃ with iron(III) chloride (FeCl₃) or boron trifluoride (BF₃), albeit with reduced yields. Additionally, solvent recovery systems in industrial setups mitigate environmental impact by recycling dichloromethane and toluene.
Chemical Reactions Analysis
Lapachol undergoes various chemical reactions, including oxidation, reduction, and substitution. For example, exposure of this compound to ozonolysis conditions results in the formation of an aldehyde at a 70% yield . This compound can also be bioactivated by P450 reductase into reactive species that promote DNA scission through the generation of superoxide anion radicals by redox cycling . Common reagents and conditions used in these reactions include molecular flexible docking studies and bioactivity assays to determine the ability of this compound to interact and inhibit specific enzymes .
Scientific Research Applications
Anticancer Properties
Mechanism of Action:
Lapachol exhibits cytotoxic effects against various cancer cell lines, including glioma and leukemia cells. Research indicates that this compound and its derivatives can induce apoptosis in tumor cells through different pathways. For instance, glycosylated derivatives of this compound have shown enhanced cytotoxicity compared to the parent compound. In a study involving HL-60 human leukemia cells, the glycosylated forms (LA4A and LA4C) demonstrated lower IC50 values (5.7 μM and 5.3 μM respectively) compared to this compound (25 μM), indicating a stronger anti-cancer effect .
Case Study: Glioma Treatment
Recent studies have highlighted this compound's effectiveness in treating malignant glioma. One study demonstrated that this compound significantly reduced the survival rate of spongioblastoma cells in vitro, outperforming the conventional drug temozolomide at certain concentrations . Additionally, the development of liposomal formulations of this compound has improved its pharmacokinetics and brain delivery, making it a promising candidate for glioma therapy .
Wound Healing
Research Findings:
this compound has been investigated for its wound healing properties, particularly in diabetic models. In a controlled study, mice treated with this compound exhibited significantly faster wound closure compared to control groups. The mechanism was linked to the upregulation of sirtuin proteins (Sirt1 and Sirt3), which play crucial roles in cellular repair and regeneration processes . The enhanced expression of growth factors such as EGF (epidermal growth factor) further supports this compound's role in promoting wound healing.
Antimicrobial Activity
Broad-Spectrum Efficacy:
this compound has demonstrated antimicrobial properties against a variety of pathogens. It exhibits activity against bacteria such as Staphylococcus aureus and Enterococcus faecalis, with minimum inhibitory concentration (MIC) values ranging from 1.56 to 25 µg/ml . Furthermore, it has shown antifungal activity comparable to conventional antifungal agents like Amphotericin B against Candida species.
Antioxidant and Other Therapeutic Effects
Oxidative Stress Management:
this compound's antioxidant properties enable it to neutralize free radicals, potentially mitigating oxidative stress-related diseases such as cardiovascular disorders and neurodegenerative diseases . Additionally, it has been explored for its analgesic effects and potential use in treating malaria and leishmaniasis due to its activity against respective parasites.
Summary Table of Applications
Mechanism of Action
The mechanisms of action underlying the observed effects of lapachol are mainly due to its capacity to interact with topoisomerases and to generate semiquinone radicals and reactive oxygen species (ROS) inside the cell . These reactive species can cause damage to cellular components, leading to the observed pharmacological effects. This compound’s antifungal activity is believed to be due to its interaction with the cellular membrane .
Comparison with Similar Compounds
Lapachol belongs to the naphthoquinone class, which includes structurally related compounds with varying pharmacological profiles. Below is a detailed comparison:
Structural Analogs of this compound
β-Lapachone
- Structure: Cyclized derivative of this compound, forming a furan-fused naphthoquinone .
- Key Differences: Enhanced redox cycling capacity due to the fused furan ring, enabling NAD(P)H:quinone oxidoreductase 1 (NQO1)-dependent reactive oxygen species (ROS) generation .
- Bioactivity: Superior antitumor activity compared to this compound, with selective cytotoxicity in NQO1-overexpressing cancers (e.g., pancreatic, lung) . Phase II clinical trials for cancer therapy, either as monotherapy or combined with DNA repair inhibitors .
- Mechanism : NQO1-mediated ROS production induces DNA damage and PARP1 hyperactivation, leading to apoptosis .
α-Lapachone
- Structure: Isomer of β-lapachone with a different quinone ring orientation .
- Key Differences : Reduced redox stability compared to β-lapachone, limiting its clinical use .
- Bioactivity: Antiparasitic activity against Trypanosoma cruzi (Chagas disease) with IC₅₀ values comparable to benznidazole . Pyridine-modified α-lapachone derivatives exhibit enhanced trypanocidal activity via oxidative stress mechanisms .
Hydroxy-Hydrothis compound
- Structure : Hydrogenated derivative of this compound with a hydroxyl group at C-2 .
- Key Differences : Saturated side chain reduces electrophilicity, altering antioxidant and electrolytic properties .
- Bioactivity :
Thiosemicarbazone and Semicarbazone Derivatives
- Structure: this compound conjugated with thiosemicarbazide or semicarbazide groups at the quinone carbonyl .
- Key Differences : Enhanced hydrogen-bonding capacity and metal chelation properties .
- Bioactivity :
Isothis compound Acetate
- Structure : Acetylated derivative of isothis compound, a positional isomer of this compound .
- Key Differences : Increased lipophilicity and membrane permeability .
This compound-Fe(II) Complex
- Structure: Coordination complex with Fe(II) via phenolic and carbonyl oxygens .
- Key Differences : Metal coordination enhances stability and redox activity .
- Bioactivity :
Comparative Analysis of Key Properties
Compound | Source/Modification | Key Bioactivity | Mechanism of Action | Advantages Over this compound | Limitations |
---|---|---|---|---|---|
This compound | Natural (Bignoniaceae) | Anti-inflammatory, antimicrobial | Sirtuin activation, cytokine inhibition | Broad-spectrum activity | Dose-dependent toxicity, poor solubility |
β-Lapachone | Cyclized derivative | Antitumor | NQO1-dependent ROS generation | Clinical efficacy, target specificity | NQO1 dependency limits applicability |
Thiosemicarbazone | Synthetic modification | Antifungal | Membrane disruption, chelation | Lower toxicity, enhanced activity | Limited in vivo data |
Isothis compound Acetate | Acetylation | Antileishmanial | Mitochondrial dysfunction | High potency | Narrow therapeutic window |
Research Findings and Clinical Relevance
- Anticancer Activity : β-Lapachone’s clinical progression highlights its superiority over this compound in targeting NQO1-rich tumors . This compound derivatives like VI (modified with hydrophobic groups) show promise against viral targets like SARS-CoV-2 Nsp9 .
- Anti-Inflammatory Effects : this compound reduces ear edema by 40–60% in murine models, comparable to diclofenac, via downregulation of VEGF and PDGF .
- Antimicrobial Potency : Thiosemicarbazone derivatives inhibit Staphylococcus aureus at 0.10 µmol/mL, outperforming this compound’s MIC of 0.50 µmol/mL .
Biological Activity
Lapachol, a naturally occurring naphthoquinone derivative primarily isolated from the bark of Tabebuia avellanedae and other species, has garnered significant attention for its diverse biological activities. This article explores the compound's pharmacological effects, including its antimicrobial, anticancer, anti-inflammatory, and antimalarial properties, supported by various research findings and case studies.
Chemical Structure and Properties
This compound's chemical structure is characterized by a naphthoquinone framework, which is pivotal to its biological activity. The presence of hydroxyl groups enhances its reactivity and interaction with biological targets.
Antimicrobial Activity
This compound exhibits notable antimicrobial properties against a range of pathogens.
- Minimum Inhibitory Concentrations (MICs) :
Table 1: Antimicrobial Activity of this compound and Derivatives
Pathogen | MIC (µmol/mL) | Compound Type |
---|---|---|
Staphylococcus aureus | 0.10 | This compound |
Enterococcus faecalis | 0.05 | This compound |
Cryptococcus gattii | 0.20 | This compound |
Paracoccidioides brasiliensis | 0.01-0.10 | Thiosemicarbazone Derivative |
Anticancer Activity
Research indicates that this compound has significant cytotoxic effects against various cancer cell lines, including A549 human lung cancer cells.
- Cytotoxicity :
Table 2: Cytotoxic Effects of this compound
Cell Line | IC50 (mM) | Condition |
---|---|---|
A549 (lung cancer) | mM | Aerobic |
A549 (lung cancer) | mM | Hypoxic |
Anti-inflammatory Properties
This compound has been reported to possess anti-inflammatory effects, which contribute to its therapeutic potential in treating inflammatory diseases. The mechanisms may involve the inhibition of pro-inflammatory cytokines and modulation of immune responses.
Antimalarial Activity
This compound has also been explored for its antimalarial properties, particularly in the synthesis of derivatives that enhance activity against Plasmodium species.
- Research Findings : Studies have indicated that this compound can be converted into more effective antimalarial agents through chemical modifications .
Case Studies
- Thiosemicarbazone Derivatives : A study synthesized thiosemicarbazone derivatives of this compound, which exhibited enhanced antimicrobial activity against multidrug-resistant strains of bacteria .
- Comparative Studies : In bioassays against Aedes aegypti, this compound showed a lower LD50 value compared to its amine derivatives, emphasizing the importance of functional groups in determining biological activity .
Q & A
Basic Research Questions
Q. What are the primary mechanisms underlying Lapachol's anticancer activity?
this compound targets pyruvate kinase M2 (PKM2), a key glycolytic enzyme, by binding to its regulatory domain (free energy: -9.34 kcal/mol) and inhibiting glycolysis in cancer cells . It also disrupts mitochondrial electron transport by interfering with cytochromes b and c, generating reactive oxygen species (ROS) . Additionally, this compound inhibits ribosomal protein S6 kinase 2 (RSK2), suppressing growth and inducing apoptosis in esophageal squamous cell carcinoma (ESCC) cells via caspase-3/7 activation and BAX upregulation .
Q. What is the antimicrobial spectrum of this compound?
this compound exhibits broad-spectrum activity against bacteria (e.g., Helicobacter pylori, Staphylococcus spp., MIC: 1.56–25 mg/mL), fungi (e.g., Candida albicans, comparable to amphotericin B), and viruses (HSV-1, HIV-1, EBV) . Its antifungal action involves membrane disruption, while antiviral effects stem from polymerase inhibition and ROS generation .
Q. What pharmacokinetic challenges limit this compound's clinical application?
Poor aqueous solubility, rapid elimination (brain-to-plasma AUC ratio: 0.028%), and low bioavailability hinder its efficacy. Blood-brain barrier (BBB) penetration is minimal, and high doses cause hepatotoxicity (elevated Gamma GT, GPT) . Validated UPLC-MS/MS methods (LLOQ: 0.5 ng/mL) are used to quantify plasma and brain distribution .
Q. How does this compound exert its antimalarial effects?
this compound inhibits Plasmodium respiration by disrupting electron transport between cytochromes b and c (74% oxygen absorption inhibition in P. knowlesi). Computational studies show preferential binding to P. falciparum HSP70-x (ΔG: -8.2 kcal/mol) over PfHSP70-1, suggesting species-specific targeting .
Advanced Research Questions
Q. How can researchers address contradictory data on this compound's anticancer efficacy?
Historical NCI studies noted toxicity at high doses (LD₅₀: 0.62 g/kg in rats), but derivatives like β-lapachone show improved safety (LD₅₀: 0.16 g/kg) . Discrepancies arise from purity variations and metabolic plasticity in cancer models. Orthotopic glioma studies reveal tumor volume reduction (40–60%) without weight loss, highlighting context-dependent efficacy .
Q. What experimental strategies optimize this compound's bioavailability?
Nano-liposomes (85.92 nm particle size, 92.52% encapsulation) prolong circulation (t₁/₂: 12.4 vs. 3.2 hours for free this compound) and enhance brain uptake. Orthogonal design (entrapment efficiency as index) and in vitro release profiling validate sustained delivery .
Q. How do this compound combination therapies enhance efficacy?
Synergy with 2,4-dinitrophenol (DNP) increases melanoma apoptosis (20% vs. 13% alone) via mitochondrial membrane depolarization . For tuberculosis, co-administration with N-acetylcysteine (NAC) improves selectivity (SI: 12.3 to 33.2) at acidic pH .
Q. What structural features of this compound influence its molecular targets?
Molecular docking (AutoDock 4.2) identifies PKM2 binding via 19 residues (e.g., ASN43, ARG105) with π-alkyl interactions. The naphthoquinone scaffold’s hydroxyl and prenyl groups are critical for RSK2 inhibition (IC₅₀: 2.5 µM in ESCC) .
Q. How do in vitro and in vivo models differ in assessing this compound's antimetastatic effects?
In vitro, this compound (400 µg/mL) alters HeLa cell protein profiles without reducing viability. In vivo, it inhibits CAM model invasiveness (50–70%) but requires nanoparticle formulations to sustain therapeutic concentrations .
Q. What analytical methods validate this compound quantification in plant extracts?
HPTLC-UV (toluene:ethyl acetate:acetic acid, 8.5:1.5:0.02) achieves linear quantification (10–130 µg, R²=0.9973) with LOD/LOQ of 0.028/0.086 µg. RP-HPLC standardizes extracts (2.04% w/w this compound in Radermachera xylocarpa) .
Properties
IUPAC Name |
4-hydroxy-3-(3-methylbut-2-enyl)naphthalene-1,2-dione | |
---|---|---|
Source | PubChem | |
URL | https://pubchem.ncbi.nlm.nih.gov | |
Description | Data deposited in or computed by PubChem | |
InChI |
InChI=1S/C15H14O3/c1-9(2)7-8-12-13(16)10-5-3-4-6-11(10)14(17)15(12)18/h3-7,16H,8H2,1-2H3 | |
Source | PubChem | |
URL | https://pubchem.ncbi.nlm.nih.gov | |
Description | Data deposited in or computed by PubChem | |
InChI Key |
CWPGNVFCJOPXFB-UHFFFAOYSA-N | |
Source | PubChem | |
URL | https://pubchem.ncbi.nlm.nih.gov | |
Description | Data deposited in or computed by PubChem | |
Canonical SMILES |
CC(=CCC1=C(C2=CC=CC=C2C(=O)C1=O)O)C | |
Source | PubChem | |
URL | https://pubchem.ncbi.nlm.nih.gov | |
Description | Data deposited in or computed by PubChem | |
Molecular Formula |
C15H14O3 | |
Source | PubChem | |
URL | https://pubchem.ncbi.nlm.nih.gov | |
Description | Data deposited in or computed by PubChem | |
DSSTOX Substance ID |
DTXSID6049430 | |
Record name | Lapachol | |
Source | EPA DSSTox | |
URL | https://comptox.epa.gov/dashboard/DTXSID6049430 | |
Description | DSSTox provides a high quality public chemistry resource for supporting improved predictive toxicology. | |
Molecular Weight |
242.27 g/mol | |
Source | PubChem | |
URL | https://pubchem.ncbi.nlm.nih.gov | |
Description | Data deposited in or computed by PubChem | |
CAS No. |
84-79-7 | |
Record name | Lapachol | |
Source | ChemIDplus | |
URL | https://pubchem.ncbi.nlm.nih.gov/substance/?source=chemidplus&sourceid=0000084797 | |
Description | ChemIDplus is a free, web search system that provides access to the structure and nomenclature authority files used for the identification of chemical substances cited in National Library of Medicine (NLM) databases, including the TOXNET system. | |
Record name | lapachol | |
Source | DTP/NCI | |
URL | https://dtp.cancer.gov/dtpstandard/servlet/dwindex?searchtype=NSC&outputformat=html&searchlist=11905 | |
Description | The NCI Development Therapeutics Program (DTP) provides services and resources to the academic and private-sector research communities worldwide to facilitate the discovery and development of new cancer therapeutic agents. | |
Explanation | Unless otherwise indicated, all text within NCI products is free of copyright and may be reused without our permission. Credit the National Cancer Institute as the source. | |
Record name | Lapachol | |
Source | EPA DSSTox | |
URL | https://comptox.epa.gov/dashboard/DTXSID6049430 | |
Description | DSSTox provides a high quality public chemistry resource for supporting improved predictive toxicology. | |
Record name | 2-hydroxy-3-(3-methylbut-2-enyl)-1,4-naphthoquinone | |
Source | European Chemicals Agency (ECHA) | |
URL | https://echa.europa.eu/substance-information/-/substanceinfo/100.001.421 | |
Description | The European Chemicals Agency (ECHA) is an agency of the European Union which is the driving force among regulatory authorities in implementing the EU's groundbreaking chemicals legislation for the benefit of human health and the environment as well as for innovation and competitiveness. | |
Explanation | Use of the information, documents and data from the ECHA website is subject to the terms and conditions of this Legal Notice, and subject to other binding limitations provided for under applicable law, the information, documents and data made available on the ECHA website may be reproduced, distributed and/or used, totally or in part, for non-commercial purposes provided that ECHA is acknowledged as the source: "Source: European Chemicals Agency, http://echa.europa.eu/". Such acknowledgement must be included in each copy of the material. ECHA permits and encourages organisations and individuals to create links to the ECHA website under the following cumulative conditions: Links can only be made to webpages that provide a link to the Legal Notice page. | |
Record name | LAPACHOL | |
Source | FDA Global Substance Registration System (GSRS) | |
URL | https://gsrs.ncats.nih.gov/ginas/app/beta/substances/B221938VB6 | |
Description | The FDA Global Substance Registration System (GSRS) enables the efficient and accurate exchange of information on what substances are in regulated products. Instead of relying on names, which vary across regulatory domains, countries, and regions, the GSRS knowledge base makes it possible for substances to be defined by standardized, scientific descriptions. | |
Explanation | Unless otherwise noted, the contents of the FDA website (www.fda.gov), both text and graphics, are not copyrighted. They are in the public domain and may be republished, reprinted and otherwise used freely by anyone without the need to obtain permission from FDA. Credit to the U.S. Food and Drug Administration as the source is appreciated but not required. | |
Retrosynthesis Analysis
AI-Powered Synthesis Planning: Our tool employs the Template_relevance Pistachio, Template_relevance Bkms_metabolic, Template_relevance Pistachio_ringbreaker, Template_relevance Reaxys, Template_relevance Reaxys_biocatalysis model, leveraging a vast database of chemical reactions to predict feasible synthetic routes.
One-Step Synthesis Focus: Specifically designed for one-step synthesis, it provides concise and direct routes for your target compounds, streamlining the synthesis process.
Accurate Predictions: Utilizing the extensive PISTACHIO, BKMS_METABOLIC, PISTACHIO_RINGBREAKER, REAXYS, REAXYS_BIOCATALYSIS database, our tool offers high-accuracy predictions, reflecting the latest in chemical research and data.
Strategy Settings
Precursor scoring | Relevance Heuristic |
---|---|
Min. plausibility | 0.01 |
Model | Template_relevance |
Template Set | Pistachio/Bkms_metabolic/Pistachio_ringbreaker/Reaxys/Reaxys_biocatalysis |
Top-N result to add to graph | 6 |
Feasible Synthetic Routes
Disclaimer and Information on In-Vitro Research Products
Please be aware that all articles and product information presented on BenchChem are intended solely for informational purposes. The products available for purchase on BenchChem are specifically designed for in-vitro studies, which are conducted outside of living organisms. In-vitro studies, derived from the Latin term "in glass," involve experiments performed in controlled laboratory settings using cells or tissues. It is important to note that these products are not categorized as medicines or drugs, and they have not received approval from the FDA for the prevention, treatment, or cure of any medical condition, ailment, or disease. We must emphasize that any form of bodily introduction of these products into humans or animals is strictly prohibited by law. It is essential to adhere to these guidelines to ensure compliance with legal and ethical standards in research and experimentation.