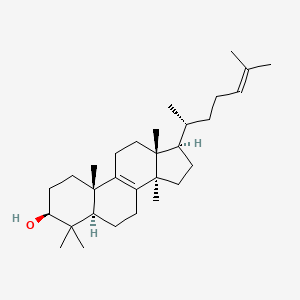
Lanosterol
Overview
Description
Lanosterol is a tetracyclic triterpenoid and serves as a crucial intermediate in the biosynthesis of steroids in animals and fungi. It is the compound from which all animal and fungal steroids are derived, while plant steroids are produced via cycloartenol . This compound plays a significant role in the cholesterol biosynthesis pathway, making it a vital molecule in cellular metabolism and physiology .
Mechanism of Action
Target of Action: Lanosterol Synthase
This compound primarily targets This compound synthase (LSS) , an enzyme involved in cholesterol biosynthesis. LSS catalyzes the cyclization of (S)-2,3-oxidosqualene to this compound, a critical step in sterol nucleus formation . This compound synthase plays a pivotal role in the production of cholesterol and other sterols.
Pharmacokinetics: ADME Properties
- Absorption : this compound’s absorption depends on its route of administration. For instance, this compound eye drops penetrate the lens of the eye, where they interact with cataract proteins .
Result of Action: Cellular Effects
This compound’s action has significant implications: - Cholesterol Homeostasis : By participating in cholesterol synthesis, this compound influences cellular cholesterol levels, which impact membrane fluidity, signaling, and lipid rafts. - Cataract Treatment : this compound eye drops may help break down protein clumps in cataracts, potentially restoring lens clarity .
Action Environment: Environmental Factors
This compound’s efficacy and stability can be influenced by environmental conditions, such as temperature, pH, and oxidative stress. These factors may affect its ability to interact with LSS and participate in cholesterol biosynthesis.
: DrugBank: this compound : This compound Eye Drops: A New Solution for Cataracts
Biochemical Analysis
Biochemical Properties
Lanosterol is involved in several biochemical reactions, primarily in the cholesterol biosynthesis pathway. It is synthesized from squalene through the action of this compound synthase. This compound interacts with various enzymes, including this compound 14α-demethylase (CYP51A1), which catalyzes the demethylation of this compound to produce cholesterol . This interaction is crucial for the conversion of this compound into cholesterol, a vital component of cell membranes and a precursor for steroid hormones and bile acids .
Cellular Effects
This compound has been shown to influence various cellular processes. It induces mitochondrial uncoupling and protects dopaminergic neurons from cell death in models of Parkinson’s disease . This compound also modulates the synthesis of 24,25-epoxysterol, which promotes the formation of oligodendrocytes, cells responsible for myelination in the central nervous system . Additionally, this compound has been found to accumulate in macrophages upon activation of Toll-like receptor 4, indicating its role in innate immune responses .
Molecular Mechanism
At the molecular level, this compound exerts its effects through several mechanisms. It binds to and inhibits this compound 14α-demethylase (CYP51A1), a key enzyme in the cholesterol biosynthesis pathway . This inhibition leads to the accumulation of this compound and other sterol intermediates, which can have various downstream effects on cellular metabolism and function. This compound also influences gene expression by modulating the activity of transcription factors involved in cholesterol homeostasis .
Temporal Effects in Laboratory Settings
In laboratory settings, the effects of this compound have been observed to change over time. For instance, in studies involving cataractous lenses of cynomolgus monkeys, this compound showed a short-term reversal effect on cataract severity, with its concentration in aqueous humor peaking within the first two weeks and gradually decreasing thereafter . This suggests that this compound’s effects are time-dependent and may diminish over prolonged periods.
Dosage Effects in Animal Models
The effects of this compound vary with different dosages in animal models. In studies involving zebrafish and dogs, this compound eye drops were found to reduce cataract severity and improve lens transparency The efficacy of this compound is dose-dependent, with higher doses showing more pronounced effects
Metabolic Pathways
. This pathway involves several enzymes, including hydroxymethylglutaryl-CoA reductase, squalene synthase, and this compound synthase. This compound is converted to cholesterol through a series of enzymatic reactions, with this compound 14α-demethylase playing a critical role in this process . The pathway also produces other important metabolites, such as steroid hormones and bile acids .
Transport and Distribution
This compound is transported and distributed within cells and tissues through various mechanisms. It is synthesized in the endoplasmic reticulum and transported to other cellular compartments where it undergoes further processing . This compound interacts with sterol transporters and binding proteins that facilitate its movement within the cell. Its distribution is crucial for maintaining cellular cholesterol homeostasis and ensuring the proper functioning of cell membranes .
Subcellular Localization
This compound is primarily localized in the endoplasmic reticulum, where it is synthesized and undergoes initial processing . It can also be found in other cellular compartments, such as mitochondria, where it may exert additional effects on cellular metabolism . The subcellular localization of this compound is influenced by various factors, including targeting signals and post-translational modifications that direct it to specific compartments or organelles .
Preparation Methods
Synthetic Routes and Reaction Conditions: Lanosterol can be synthesized through the cyclization of squalene, a process catalyzed by the enzyme this compound synthase. The biosynthesis involves the conversion of two molecules of farnesyl pyrophosphate into squalene, which is then oxidized to 2,3-oxidosqualene. This intermediate undergoes a series of cyclization reactions to form this compound .
Industrial Production Methods: Industrial production of this compound often involves extraction from natural sources such as lanolin, a waxy substance derived from sheep’s wool. The crude this compound is then purified using techniques like high-performance liquid chromatography (HPLC) to achieve high purity levels .
Chemical Reactions Analysis
Types of Reactions: Lanosterol undergoes various chemical reactions, including:
Oxidation: this compound can be oxidized to form different derivatives, such as 4-methyl-4-carboxyzymosterone.
Reduction: Reduction reactions can convert this compound into dihydrothis compound.
Substitution: this compound can participate in substitution reactions, particularly in the presence of strong acids or bases.
Common Reagents and Conditions:
Oxidizing Agents: Potassium permanganate (KMnO4), chromium trioxide (CrO3).
Reducing Agents: Sodium borohydride (NaBH4), lithium aluminum hydride (LiAlH4).
Catalysts: Enzymes like this compound synthase and squalene monooxygenase.
Major Products:
Cholesterol: this compound is a precursor to cholesterol through a series of demethylation, reduction, and isomerization steps.
Steroid Hormones: this compound derivatives are involved in the synthesis of various steroid hormones.
Scientific Research Applications
Chemistry: Lanosterol serves as a model compound for studying triterpenoid biosynthesis and enzymatic cyclization processes.
Biology: It is a key intermediate in the biosynthesis of sterols and steroid hormones, making it essential for cellular function and metabolism.
Medicine: this compound has shown promise in the treatment of cataracts.
Industry: this compound is used in the production of cosmetics and pharmaceuticals due to its emollient and stabilizing properties.
Comparison with Similar Compounds
Lanosterol is unique among triterpenoids due to its role as a precursor to cholesterol and other steroids. Similar compounds include:
Cycloartenol: The plant equivalent of this compound, serving as a precursor to plant steroids.
Dihydrothis compound: A reduced form of this compound, often used in studies of sterol metabolism.
This compound’s unique position in the biosynthesis of cholesterol and its potential therapeutic applications make it a compound of significant interest in both scientific research and industrial applications.
Biological Activity
Lanosterol, a key intermediate in the biosynthesis of cholesterol, has garnered attention for its diverse biological activities, particularly in the context of immunity, neuroprotection, and cholesterol metabolism. This article delves into the biological activity of this compound, highlighting recent research findings and case studies that elucidate its mechanisms and potential therapeutic applications.
Overview of this compound
This compound is a tetracyclic triterpenoid synthesized from squalene in the cholesterol biosynthetic pathway. Its structure allows it to partake in various biological processes, influencing cellular functions and immune responses.
Immune Modulation
Recent studies have demonstrated that this compound plays a significant role in modulating immune responses, particularly in macrophages (MΦs).
- Macrophage Activation : this compound accumulation in macrophages enhances their phagocytic activity while reducing the secretion of pro-inflammatory cytokines. This dual action promotes a more effective antimicrobial response and reduces the risk of excessive inflammation during infections .
- Mechanistic Insights : The accumulation of this compound is linked to the downregulation of this compound 14α-demethylase (Cyp51A1) in TLR4-activated macrophages. This downregulation appears to be mediated by type I interferon signaling pathways, leading to increased survival rates in models of endotoxemic shock and improved bacterial clearance during infections with pathogens like Listeria monocytogenes .
Neuroprotective Effects
This compound has also been investigated for its neuroprotective properties.
- Neuroprotection Studies : In pharmacological screening, this compound analogs were identified as compounds that could potentially protect neurons from damage during ischemic events. These compounds were shown to reduce infarction size and neuronal damage when administered post-injury in animal models .
- Mechanisms : The neuroprotective effects are thought to arise from this compound's ability to modulate cholesterol metabolism within neuronal cells. By influencing the levels of cholesterol and its precursors, this compound may help maintain membrane integrity and promote neuronal survival under stress conditions .
Cholesterol Biosynthesis Inhibition
This compound's role as a precursor in cholesterol biosynthesis has led to the exploration of its analogs as potential therapeutic agents for hypercholesterolemia.
- Dual-Action Inhibitors : Research has focused on developing this compound analogs that inhibit key enzymes involved in cholesterol synthesis, such as 3-hydroxy-3-methylglutaryl coenzyme A reductase (HMGR). These analogs can effectively lower cholesterol levels without suppressing LDL receptor activity, which is crucial for maintaining lipid homeostasis .
- Clinical Implications : The ability of these analogs to selectively inhibit HMGR while preserving LDL receptor function suggests their potential as safer alternatives to traditional statin therapies .
Summary of Research Findings
The following table summarizes key findings from recent studies on this compound's biological activities:
Case Studies
- Endotoxemic Shock Model : In a controlled study involving mice subjected to endotoxemic shock, those treated with ketoconazole (which increases intracellular this compound) exhibited significantly improved survival rates compared to untreated controls. This was attributed to reduced cytokine secretion and enhanced macrophage function .
- Ischemic Stroke Model : In an ischemic stroke model using rats, administration of this compound analogs resulted in a marked reduction in infarction size and improved functional recovery post-injury, highlighting its potential therapeutic benefits in neurodegenerative conditions .
Properties
IUPAC Name |
(3S,5R,10S,13R,14R,17R)-4,4,10,13,14-pentamethyl-17-[(2R)-6-methylhept-5-en-2-yl]-2,3,5,6,7,11,12,15,16,17-decahydro-1H-cyclopenta[a]phenanthren-3-ol | |
---|---|---|
Source | PubChem | |
URL | https://pubchem.ncbi.nlm.nih.gov | |
Description | Data deposited in or computed by PubChem | |
InChI |
InChI=1S/C30H50O/c1-20(2)10-9-11-21(3)22-14-18-30(8)24-12-13-25-27(4,5)26(31)16-17-28(25,6)23(24)15-19-29(22,30)7/h10,21-22,25-26,31H,9,11-19H2,1-8H3/t21-,22-,25+,26+,28-,29-,30+/m1/s1 | |
Source | PubChem | |
URL | https://pubchem.ncbi.nlm.nih.gov | |
Description | Data deposited in or computed by PubChem | |
InChI Key |
CAHGCLMLTWQZNJ-BQNIITSRSA-N | |
Source | PubChem | |
URL | https://pubchem.ncbi.nlm.nih.gov | |
Description | Data deposited in or computed by PubChem | |
Canonical SMILES |
CC(CCC=C(C)C)C1CCC2(C1(CCC3=C2CCC4C3(CCC(C4(C)C)O)C)C)C | |
Source | PubChem | |
URL | https://pubchem.ncbi.nlm.nih.gov | |
Description | Data deposited in or computed by PubChem | |
Isomeric SMILES |
C[C@H](CCC=C(C)C)[C@H]1CC[C@@]2([C@@]1(CCC3=C2CC[C@@H]4[C@@]3(CC[C@@H](C4(C)C)O)C)C)C | |
Source | PubChem | |
URL | https://pubchem.ncbi.nlm.nih.gov | |
Description | Data deposited in or computed by PubChem | |
Molecular Formula |
C30H50O | |
Source | PubChem | |
URL | https://pubchem.ncbi.nlm.nih.gov | |
Description | Data deposited in or computed by PubChem | |
DSSTOX Substance ID |
DTXSID1040744 | |
Record name | Lanosterol | |
Source | EPA DSSTox | |
URL | https://comptox.epa.gov/dashboard/DTXSID1040744 | |
Description | DSSTox provides a high quality public chemistry resource for supporting improved predictive toxicology. | |
Molecular Weight |
426.7 g/mol | |
Source | PubChem | |
URL | https://pubchem.ncbi.nlm.nih.gov | |
Description | Data deposited in or computed by PubChem | |
Physical Description |
Solid | |
Record name | Lanosterol | |
Source | Human Metabolome Database (HMDB) | |
URL | http://www.hmdb.ca/metabolites/HMDB0001251 | |
Description | The Human Metabolome Database (HMDB) is a freely available electronic database containing detailed information about small molecule metabolites found in the human body. | |
Explanation | HMDB is offered to the public as a freely available resource. Use and re-distribution of the data, in whole or in part, for commercial purposes requires explicit permission of the authors and explicit acknowledgment of the source material (HMDB) and the original publication (see the HMDB citing page). We ask that users who download significant portions of the database cite the HMDB paper in any resulting publications. | |
CAS No. |
79-63-0 | |
Record name | Lanosterol | |
Source | CAS Common Chemistry | |
URL | https://commonchemistry.cas.org/detail?cas_rn=79-63-0 | |
Description | CAS Common Chemistry is an open community resource for accessing chemical information. Nearly 500,000 chemical substances from CAS REGISTRY cover areas of community interest, including common and frequently regulated chemicals, and those relevant to high school and undergraduate chemistry classes. This chemical information, curated by our expert scientists, is provided in alignment with our mission as a division of the American Chemical Society. | |
Explanation | The data from CAS Common Chemistry is provided under a CC-BY-NC 4.0 license, unless otherwise stated. | |
Record name | Lanosterol | |
Source | ChemIDplus | |
URL | https://pubchem.ncbi.nlm.nih.gov/substance/?source=chemidplus&sourceid=0000079630 | |
Description | ChemIDplus is a free, web search system that provides access to the structure and nomenclature authority files used for the identification of chemical substances cited in National Library of Medicine (NLM) databases, including the TOXNET system. | |
Record name | Lanosterol | |
Source | DrugBank | |
URL | https://www.drugbank.ca/drugs/DB03696 | |
Description | The DrugBank database is a unique bioinformatics and cheminformatics resource that combines detailed drug (i.e. chemical, pharmacological and pharmaceutical) data with comprehensive drug target (i.e. sequence, structure, and pathway) information. | |
Explanation | Creative Common's Attribution-NonCommercial 4.0 International License (http://creativecommons.org/licenses/by-nc/4.0/legalcode) | |
Record name | lanosterol | |
Source | DTP/NCI | |
URL | https://dtp.cancer.gov/dtpstandard/servlet/dwindex?searchtype=NSC&outputformat=html&searchlist=60677 | |
Description | The NCI Development Therapeutics Program (DTP) provides services and resources to the academic and private-sector research communities worldwide to facilitate the discovery and development of new cancer therapeutic agents. | |
Explanation | Unless otherwise indicated, all text within NCI products is free of copyright and may be reused without our permission. Credit the National Cancer Institute as the source. | |
Record name | Lanosterol | |
Source | EPA DSSTox | |
URL | https://comptox.epa.gov/dashboard/DTXSID1040744 | |
Description | DSSTox provides a high quality public chemistry resource for supporting improved predictive toxicology. | |
Record name | Lanosterol | |
Source | European Chemicals Agency (ECHA) | |
URL | https://echa.europa.eu/substance-information/-/substanceinfo/100.001.105 | |
Description | The European Chemicals Agency (ECHA) is an agency of the European Union which is the driving force among regulatory authorities in implementing the EU's groundbreaking chemicals legislation for the benefit of human health and the environment as well as for innovation and competitiveness. | |
Explanation | Use of the information, documents and data from the ECHA website is subject to the terms and conditions of this Legal Notice, and subject to other binding limitations provided for under applicable law, the information, documents and data made available on the ECHA website may be reproduced, distributed and/or used, totally or in part, for non-commercial purposes provided that ECHA is acknowledged as the source: "Source: European Chemicals Agency, http://echa.europa.eu/". Such acknowledgement must be included in each copy of the material. ECHA permits and encourages organisations and individuals to create links to the ECHA website under the following cumulative conditions: Links can only be made to webpages that provide a link to the Legal Notice page. | |
Record name | LANOSTEROL | |
Source | FDA Global Substance Registration System (GSRS) | |
URL | https://gsrs.ncats.nih.gov/ginas/app/beta/substances/1J05Z83K3M | |
Description | The FDA Global Substance Registration System (GSRS) enables the efficient and accurate exchange of information on what substances are in regulated products. Instead of relying on names, which vary across regulatory domains, countries, and regions, the GSRS knowledge base makes it possible for substances to be defined by standardized, scientific descriptions. | |
Explanation | Unless otherwise noted, the contents of the FDA website (www.fda.gov), both text and graphics, are not copyrighted. They are in the public domain and may be republished, reprinted and otherwise used freely by anyone without the need to obtain permission from FDA. Credit to the U.S. Food and Drug Administration as the source is appreciated but not required. | |
Record name | Lanosterol | |
Source | Human Metabolome Database (HMDB) | |
URL | http://www.hmdb.ca/metabolites/HMDB0001251 | |
Description | The Human Metabolome Database (HMDB) is a freely available electronic database containing detailed information about small molecule metabolites found in the human body. | |
Explanation | HMDB is offered to the public as a freely available resource. Use and re-distribution of the data, in whole or in part, for commercial purposes requires explicit permission of the authors and explicit acknowledgment of the source material (HMDB) and the original publication (see the HMDB citing page). We ask that users who download significant portions of the database cite the HMDB paper in any resulting publications. | |
Melting Point |
140.5 °C | |
Record name | Lanosterol | |
Source | DrugBank | |
URL | https://www.drugbank.ca/drugs/DB03696 | |
Description | The DrugBank database is a unique bioinformatics and cheminformatics resource that combines detailed drug (i.e. chemical, pharmacological and pharmaceutical) data with comprehensive drug target (i.e. sequence, structure, and pathway) information. | |
Explanation | Creative Common's Attribution-NonCommercial 4.0 International License (http://creativecommons.org/licenses/by-nc/4.0/legalcode) | |
Record name | Lanosterol | |
Source | Human Metabolome Database (HMDB) | |
URL | http://www.hmdb.ca/metabolites/HMDB0001251 | |
Description | The Human Metabolome Database (HMDB) is a freely available electronic database containing detailed information about small molecule metabolites found in the human body. | |
Explanation | HMDB is offered to the public as a freely available resource. Use and re-distribution of the data, in whole or in part, for commercial purposes requires explicit permission of the authors and explicit acknowledgment of the source material (HMDB) and the original publication (see the HMDB citing page). We ask that users who download significant portions of the database cite the HMDB paper in any resulting publications. | |
Retrosynthesis Analysis
AI-Powered Synthesis Planning: Our tool employs the Template_relevance Pistachio, Template_relevance Bkms_metabolic, Template_relevance Pistachio_ringbreaker, Template_relevance Reaxys, Template_relevance Reaxys_biocatalysis model, leveraging a vast database of chemical reactions to predict feasible synthetic routes.
One-Step Synthesis Focus: Specifically designed for one-step synthesis, it provides concise and direct routes for your target compounds, streamlining the synthesis process.
Accurate Predictions: Utilizing the extensive PISTACHIO, BKMS_METABOLIC, PISTACHIO_RINGBREAKER, REAXYS, REAXYS_BIOCATALYSIS database, our tool offers high-accuracy predictions, reflecting the latest in chemical research and data.
Strategy Settings
Precursor scoring | Relevance Heuristic |
---|---|
Min. plausibility | 0.01 |
Model | Template_relevance |
Template Set | Pistachio/Bkms_metabolic/Pistachio_ringbreaker/Reaxys/Reaxys_biocatalysis |
Top-N result to add to graph | 6 |
Feasible Synthetic Routes
Q1: How does lanosterol potentially alleviate lens opacity in age-related cortical cataracts?
A1: Research suggests that this compound might exert its effect by increasing the solubility of lens proteins, particularly α-crystallin, in the cortical region of the lens. [] Additionally, this compound may contribute to a reduction in oxidative stress levels within the lens, further supporting lens transparency. [, ]
Q2: Does this compound impact all subtypes of age-related cataracts?
A2: Studies indicate that while this compound is present in different age-related cataract subtypes, including age-related cortical cataract (ARCC), age-related nuclear cataract (ARNC), and age-related posterior subcapsular cataract (ARPSC), its levels are only correlated with the opaque degree of ARCC. [] This suggests a potentially subtype-specific effect.
Q3: What is the role of this compound synthase (LSS) in the context of this compound's effect on cataracts?
A3: LSS catalyzes the cyclization of (3S)-2,3-oxidosqualene to form this compound. [, , ] Research indicates a correlation between LSS expression and this compound levels in the lens with the severity of ARCC. [] Inhibiting LSS activity in rat lens cultures led to increased lens opacity, while this compound supplementation delayed this effect. [] This suggests a protective role of the LSS pathway and this compound in maintaining lens transparency.
Q4: How does this compound interact with human γD-crystallin (HγD-Crys) to potentially prevent cataract formation?
A4: Molecular dynamics (MD) simulation studies reveal that this compound preferentially binds to the hydrophobic dimerization interface of HγD-Crys, particularly the structured C-terminus (near residues 135-165). [] This binding is suggested to disrupt the aggregation of HγD-Crys, potentially hindering cataract formation.
Q5: What is the molecular formula and weight of this compound?
A5: The molecular formula of this compound is C30H50O, and its molecular weight is 426.72 g/mol. [, ]
Q6: Are there any spectroscopic data available for characterizing this compound?
A6: Yes, 1H and 13C NMR spectroscopic data have been used extensively to characterize this compound and its derivatives. [, ] These data provide valuable information regarding the structure and conformation of this compound.
Q7: What is the primary enzymatic reaction catalyzed by this compound synthase (LSS)?
A7: LSS catalyzes the complex cyclization of (3S)-2,3-oxidosqualene to form this compound, a crucial step in the biosynthesis of cholesterol in animals and ergosterol in fungi. [, , , ]
Q8: What is the significance of the methyl-29 group in the substrate for the LSS-catalyzed reaction?
A8: Studies using modified oxidosqualene substrates reveal that the methyl-29 group plays a critical role in the correct folding of the substrate within the LSS active site. [] This precise folding is essential for the enzyme to execute the multi-step cyclization process accurately.
Q9: How does this compound 14α-demethylase (CYP51) contribute to the biosynthesis of cholesterol?
A9: CYP51 is a cytochrome P450 enzyme that catalyzes the 14α-demethylation of this compound, an essential step in the multi-step conversion of this compound to cholesterol. [, , , , , , , , ]
Q10: How have computational methods been used to study this compound's interaction with HγD-Crys?
A10: All-atom molecular dynamics (MD) simulations and free energy perturbation (FEP) techniques have been employed to investigate the binding affinity and interaction modes of this compound with HγD-Crys. [] These simulations provide insights into the molecular mechanism by which this compound might prevent HγD-Crys aggregation.
Q11: Have there been any studies using molecular docking simulations to understand the interaction of this compound with other molecules?
A11: Yes, molecular docking simulations have been utilized to investigate the interaction of this compound with γ-oryzanol in oleogel systems. [] These studies help elucidate the structural basis of gel formation and the influence of this compound on the resulting gel properties.
Q12: How does the presence of a 3-hydroxy group influence this compound's interaction with P45014DM?
A13: Research suggests that the 3-hydroxy group of this compound is crucial for its proper orientation within the active site of P45014DM. [] Modifications at this position, such as methylation or acetylation, significantly affect the enzyme's ability to demethylate the sterol, highlighting the importance of this group for substrate recognition.
Q13: What structural features of this compound analogs are essential for their time-dependent inactivation of this compound 14α-demethylase?
A14: Studies on 15-, 32-, and 15,32-substituted this compound analogs reveal that the presence of a nuclear double bond and an abstractable 15α-proton, both essential for this compound demethylation, are also crucial for the time-dependent inactivation of the enzyme. [] This suggests that these structural features are involved in the mechanism-based inhibition of the enzyme.
Q14: What evidence supports the potential of this compound as a cataract treatment based on in vivo models?
A15: In a study using cynomolgus monkeys with cataracts, subconjunctival administration of this compound was shown to improve lens clarity, particularly in cases of cortical cataracts. [] The observed improvement correlated with an increase in this compound concentration in the aqueous humor.
Q15: Are there any human studies investigating the efficacy of this compound eye drops for cataract treatment?
A16: A study investigated the use of this compound eye drops in a human subject with a juvenile nuclear cataract. [] While the treatment was well-tolerated and didn't affect intraocular pressure, no significant cataract reversal was observed at the tested this compound concentration.
Q16: Has this compound demonstrated efficacy in other therapeutic areas?
A17: this compound has shown promising inhibitory effects against butyrylcholinesterase (BChE), acetylcholinesterase (AChE), and carbonic anhydrase I and II (CA I and II) in vitro. [] These enzymes are implicated in conditions like Alzheimer's disease and glaucoma, suggesting potential therapeutic avenues for this compound.
Disclaimer and Information on In-Vitro Research Products
Please be aware that all articles and product information presented on BenchChem are intended solely for informational purposes. The products available for purchase on BenchChem are specifically designed for in-vitro studies, which are conducted outside of living organisms. In-vitro studies, derived from the Latin term "in glass," involve experiments performed in controlled laboratory settings using cells or tissues. It is important to note that these products are not categorized as medicines or drugs, and they have not received approval from the FDA for the prevention, treatment, or cure of any medical condition, ailment, or disease. We must emphasize that any form of bodily introduction of these products into humans or animals is strictly prohibited by law. It is essential to adhere to these guidelines to ensure compliance with legal and ethical standards in research and experimentation.